Figures & data
Table 1. Platelet activation by metallic nanoparticles. Summary of metallic nanoparticles that have been shown to activate platelets (through aggregation, microscopy or flow cytometry studies) and the proposed mechanism behind the nanoparticle function. WP = studies performed using a form of washed platelets (no plasma proteins). PRP = studies performed using platelet-rich plasma (PRP; containing plasma proteins)
Table 2. Platelet activation by nonmetallic nanoparticles. Summary of nonmetallic nanoparticles that have been shown to activate platelets (through aggregation, microscopy or flow cytometry studies) and the proposed mechanism behind the nanoparticle function. WP = studies performed using a form of washed platelets (no plasma proteins). PRP = studies performed using platelet-rich plasma (PRP; containing plasma proteins)
Figure 1. Nanoparticle synthesis. a) Metallic nanoparticles (gold/platinum) can be synthesized by the nucleation process, whereby small nanoparticles (seeds) are a template for larger nanoparticle (up to 100 nm) growth. Stable nanoparticles with controlled sizes are achieved by selecting the suitable reducing agents and stabilizing agents in addition to precursor (metal salts) concentrations and temperature. Small nanoparticles are initially synthesized by the reduction of the metal precursor by sodium borohydride and stabilized by sodium citrate. Larger nanoparticles are synthesized using these small nanoparticles with ascorbic acid and sodium citrate. b) Examples of different sized platinum nanoparticles synthesized by the nucleation process. Transmission electron microscopy (TEM) images are employed to obtain the average nanoparticle diameter to generate the distribution curve. c) Functionalisation of nanoparticles by thiols to produced nanoparticle with negative or positive charges (charged = also potentially hydrophilic) and hydrophobic nanoparticles
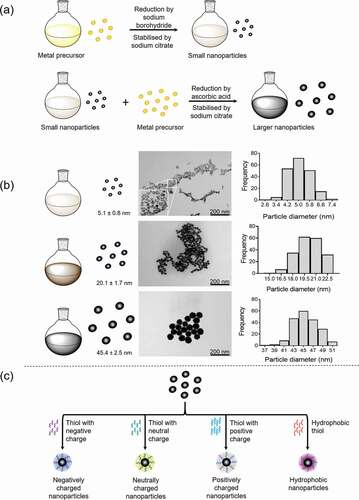
Figure 2. Schematics of potential mechanisms behind nanoparticle induced platelet activation. a) Nanoparticles are small particles (<100 nm) that can activate platelets, through different proposed mechanisms. b) Direct nanoparticle driven platelet activation. Nanoparticles could potentially bind directly to platelets, such as GPVI, leading to ITAM signaling, dimerization and clustering or through causing conformational changes in receptors, like integrin αIIbβ3 (GPIIb/IIIa) changes from an inactive confirmation to an active. c) Potential in-direct mechanisms for nanoparticle driven platelet activation. (i) nanoparticles cause general membrane disruption leading to receptor dimerization, (ii) nanoparticles crosslinking different platelet receptors, (iii) nanoparticle binding/activation leads to inhibition of protein-tyrosine phosphatases (PTPs)/ ITIM receptors, promoting platelet activation. Nanoparticles are not to scale, they are represented here are small nanoparticles (<50 nm), larger nanoparticles can be larger than the receptor height above the membrane (see Supplementary )
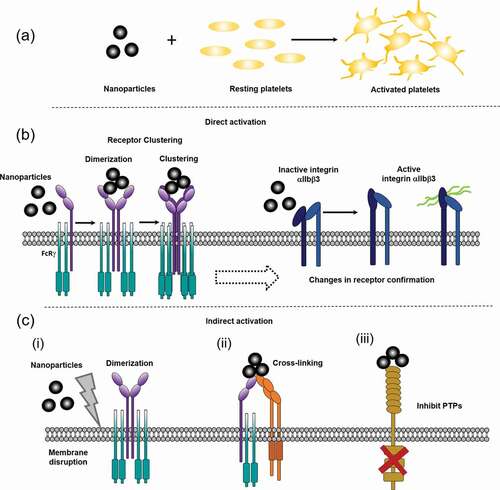
Table 3. Multiple ligands of the tyrosine kinase receptors, GPVI, CLEC-2 and PEAR1. GPVI, CLEC-2 and PEAR1 are activated by multiple ligands. Top row = endogenous ligands recognized for GPVI, CLEC-2 and PEAR1. Bottom row = exogenous ligands for GPVI, CLEC-2 and PEAR1
Figure 3. Charged DEPs and negative charged surfaces activate human platelets through a GPVI and Syk dependent manner. a) Commercially bought DEP (50 µg/mL; see Supplementary Materials for details) induced platelet aggregation is abolished in GPVI-deficient patients. (i) Representative aggregation trace of DEP and collagen (Coll; 30 µg/mL) induced aggregation in washed platelets (4x108/mL) from controls (+/+) and GPVI deficient patients (individuals homozygous (−/−) for a mutation in GP6 resulting in truncated GPVI). For more details see Supplementary Materials. (ii) Significant reduction in DEP-aggregation and collagen-induced platelet aggregation in GPVI deficient individuals. One-way ANOVA with Tukey’s multiple comparisons test, n = 3–5, ****p < .0001 to basal. (iii) Representative western blot for tyrosine phosphorylation of PLCγ2, Syk and LAT following collagen and DEP stimulated washed platelets from GPVI-deficient individuals and control individuals. b) Platelet spreading on glass (charged surface) is abolished with Syk inhibition. (i) Washed platelets (2x107/mL) spread on glass (negatively charged surface) for 30 min. Platelets were pre-incubated with 20 μM PRT-060318 (Syk inhibitor) or DMSO (vehicle) 5 min before spreading. Scale bar = 5 μm. (ii) Western blot for tyrosine phosphorylation of signaling proteins after platelets were spread on glass. Representative of 3 experiments
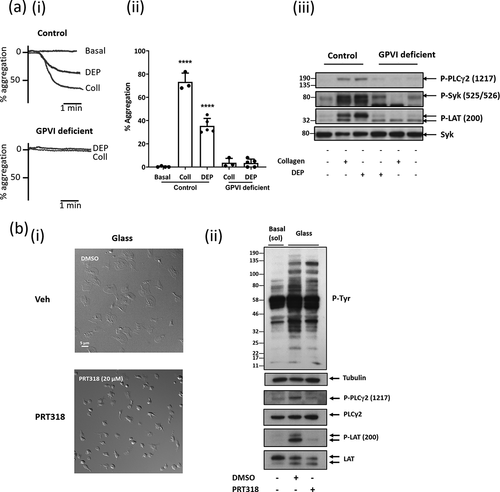
Figure 4. Charge distribution and ligand-binding interface mapped onto the surface of a) GPVI (PDB: 2GI7) and b) CLEC-2 (PDB: 2C6U) extracellular domains. Charged residues of the ligand binding interface are represented as spheres with their color corresponding to charge (red: positive, blue: negative). Additional charged residues are highlighted throughout the domains with their surface coverage displayed as a mesh
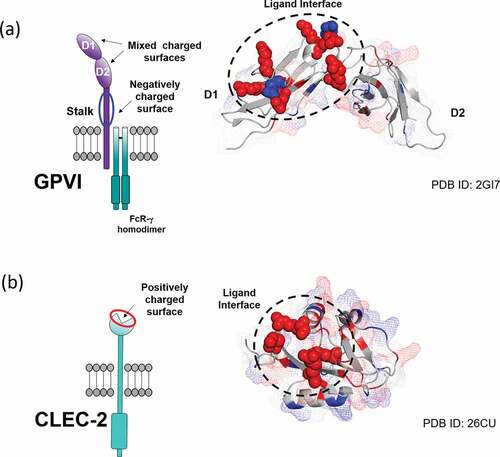