Figures & data
Figure 1. Interface of the model. The two upper square areas represent two meadows, nectar sources A and B. The dark grey areas within the nectar source areas represent flowers, while the light grey areas are nfps. The white dots (for the sake of visibility drawn bigger) are foragers searching for flowers or collecting nectar. The two lines in the lower part of the picture represent areas within the hive: the transfer zone for the foragers and the transfer zone for the storers (for the sake of visibility drawn bigger, as grey dots), where interactions between foragers and storers take place.
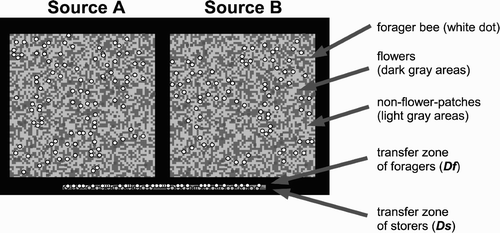
Figure 2. State diagram of a forager bee. Every behavioural state is associated with a defined metabolic rate and a defined behaviour. States associated with a high metabolic rate are shown in grey shading, those associated with a low metabolic rate in white. Abbreviations are explained in . Please keep in mind that there exists an own set of values for each source (A, B) for each transmission probability, taking place outside the colony (p lf, p af, p ff, p rc, p as, p sr).
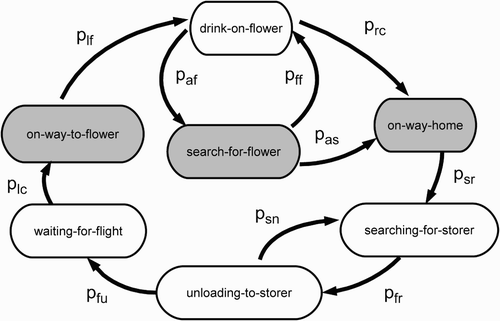
Figure 3. State diagram of a storer bee. All of the states of a storer bee are associated with the low metabolic rate, because storer bees do not leave the colony to fly out in the environment. Every state is associated with a defined behaviour. Abbreviations are explained in .
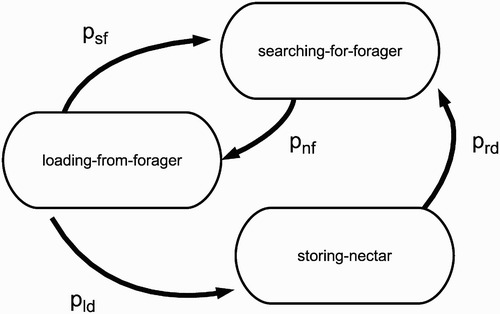
Table 1. Probabilities that a forager bee changes from one behavioural state to another.
Table 2. Probabilities that a storer changes from one behavioural state to another.
Table 3. Fixed variables used in the model.
Figure 4. Dynamics of the crop load of a forager bee during one foraging cycle as predicted by our simulation (B) and as depicted in published idealised data (Schmidt-Hempel et al. Citation1985) (A). The forager leaves the colony (a) to fly to the nectar source, lands on a flower (b), uploads the nectar (c), flies to the next flower (d), and so on. After some time the forager stops flying from flower to flower and starts to fly back to the colony (e). As soon as the forager reaches the colony (f), it unloads most of its nectar to a waiting storer bee ((g) missing in A), and then stays in the colony for Th time steps (h). The graph predicted by our model shows a foraging cycle under conditions of low nectar flow with Ma m =1 μl. The bee stops foraging before it is fully loaded (C i (t)<Ce i ).
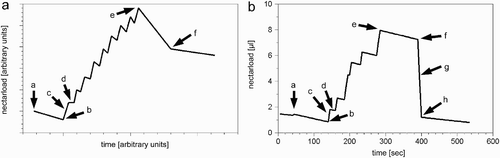
Figure 5. Dynamics of the crop load of three forager bees during one foraging cycle in environments with different flower-to-flower distances. The smaller the flower-to-flower distances are in an environment, the bigger are the nectar loads of returning forager bees.
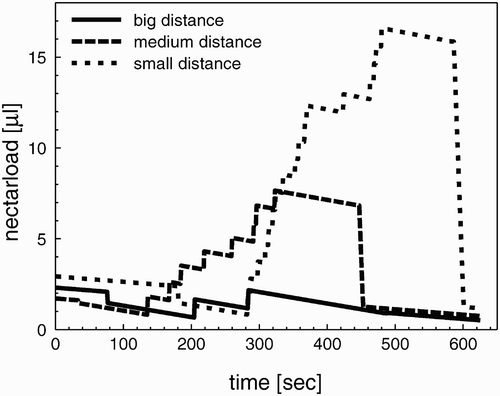
Figure 6. Nectar income of a colony with a total of 1000 foragers and receivers in relation to its forager–to-receiver ratio. Depending on the nectar flow in the environment (Ma m ) the optimum for the forager-to-receiver ratio changed.
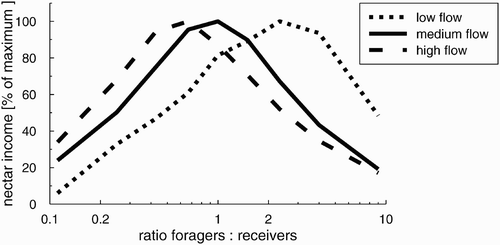
Figure 7. Crop loads of returning forager bees found in nature (A, B) and in our model (C, D). Under conditions of high nectar flow in the environment, most forager bees return to the hive with full or nearly full loads (A, C). Under conditions of low nectar flow, forager bees return with very little nectar (B, D). Graphs A and B are redrawn from (Huang and Seeley Citation2003). The larger loads in C compared with A, as well as the low variances in the crop loads of the foragers (D) compared with C are attributable to the idealised environmental situation in our simulation: all simulated flights were direct, with no wind and an entirely homogeneous environment.
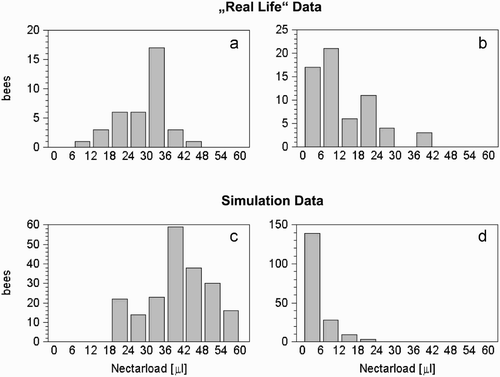
Figure 8. Changes in the percentage of multiple unloads in the group of bees flying to the source with a temporarily increased rate of nectar flow (source A) and in the group of bees flying to the source that had a constant low rate of nectar flow (source B). The grey area shows the time during which source A was treated (=high nectar flow).
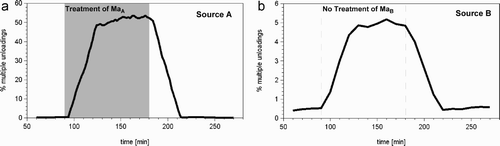
Figure 9. Changes in the duration of the searching-for-storer-period experienced by bees foraging on the treated source (A), and those foraging on the untreated source (B). During the period of high nectar flow on the treated source (90–180 min) there was a more than 10-fold longer searching time experienced by both groups of foragers, those foraging on the treated source and those foraging on the untreated source. The bold line indicates the median, the dashed lines first and third quartiles.
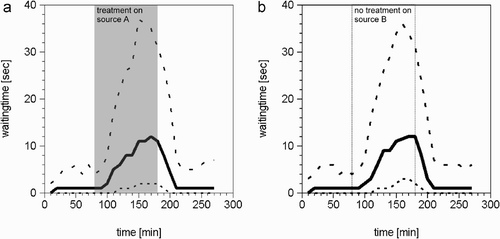
Figure 10. Differences between the crop space needed for unloading by returning foragers, and the average available crop space in storer bees per time step, in our simulations. In the experimental phase before treatment (two columns on the left), when only small amounts of nectar are brought into the colony by foragers, many storers are waiting near the hive's entrance. During the treatment (two middle columns), much larger amounts of nectar are brought into the colony by foragers, and less crop space in storers is available. After the treatment (two columns on the right), the amounts of needed and available crop space return to former levels.
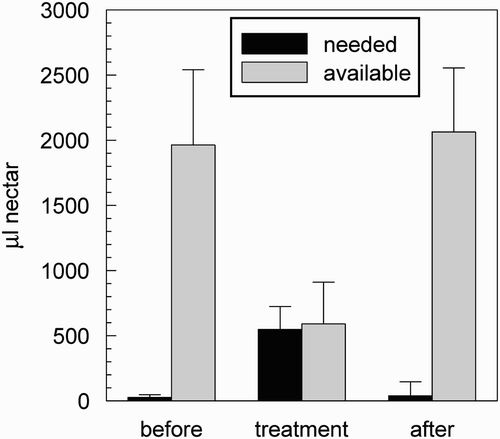
Figure 11. Comparison of the net nectar gain of a colony of 2000 bees (500 forager bees, 500 storer bees, and 1000 additional colony-bees). The colony that used the social learning gained about 20.1% more nectar than a colony without social learning. (Bars indicate mean values, whiskers indicate standard deviation. n=10.)
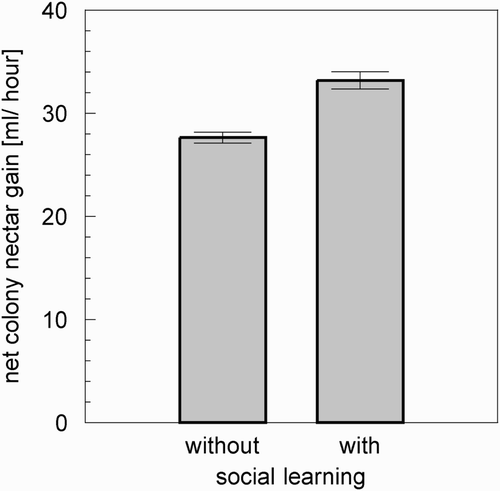
Figure 12. Scheme of the flow of information about the environmental situation between two groups of foragers via the nectar unloading mechanism. Under conditions of the coexistence of a source with high nectar flow and a source of low nectar flow, the resulting high loads of the nectar receivers lead to a high waiting time experienced by both the foragers returning from the source with the high nectar flow, and the foragers returning from the source with the low nectar flow. The arrows in the diagram symbolise causal influences.
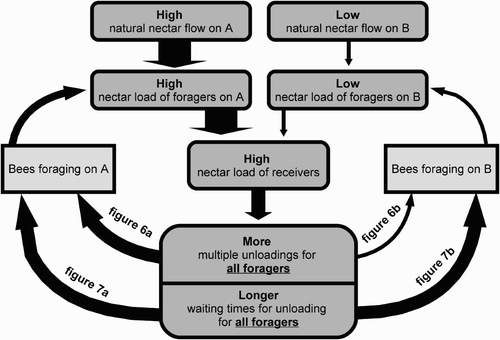