Figures & data
Table 1. Properties of radium isotopes in radiation dosimetry.
Figure 2. Different areas of lung give rise to different types of lung cancer. For example, squamous cell carcinoma (SCC) occurs mostly in large bronchi, while adenocarcinomas (ADC) affect distal lung. Radium radionuclides that emit alpha and beta particles cause ionization of biological molecules within a spherical volume that changes over time with the decay progression. The position of radium inside the lung, however, depends on the chemical form and size distribution of particulate matter that contains radium. Consequently, DNA damage resulting in mutations occurs in different areas of the lung depending on radionuclide distribution. Therefore, placement of the same radionuclide close to cells lining large vs. small and distal lung airways will result in development of different cancer types. In other words, physical objects that regulate radionuclide distribution and biological properties of the targeted cells are more important for determination of cancer type than the radionuclide itself.
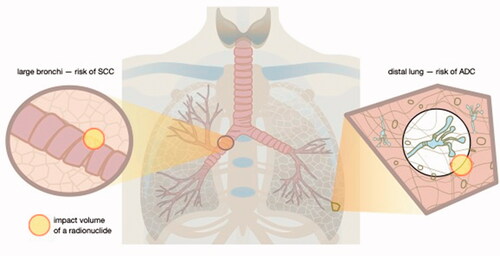
Figure 3. The anatomical location and the total magnitude of radioactivity of radium and its various progeny is depicted following three intake scenarios: (top row) inhalation of the radioisotope 226Ra, (middle row) ingestion of the radioisotope 226Ra, and (bottom row) injection of the radioisotope 223Ra. For each scenario, we show graphically the biodistribution of total activity (parent plus ingrown daughter radionuclides) at three time points since the intake (at t = 0). The colored scale is normalized to 100% which represents a unit intake (say 1 Bq) of the radium isotope by either inhalation, ingestion, or injection. We note that with in-situ decay time and biological excretion, the displayed total activity can fall below the intake value (percentages below 100%), but in other cases, the accumulation of multiple short-lived decay products (radioactive progeny) will give us a total body activity (across all radioisotopes) that exceeds the intake value (percentages above 100%).
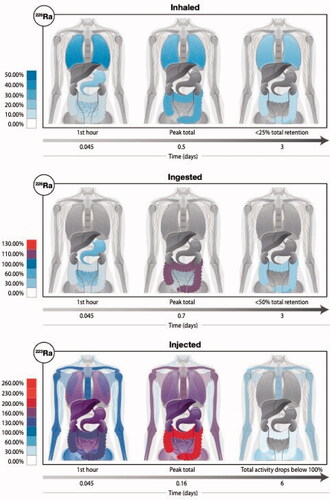
Figure 4. Mechanisms of action. The current school of thought suggests that there is enough alpha particle damage to cause apoptosis in a few keratinocytes or other epithelial cells, which in turn get phagocytosed by Langerhans cells (LC) or similar antigen presenting cells that migrate to the lymph nodes where they can modulate the immune balance systemically away from the pro-inflammatory Th1 bias (Deetjen et al. Citation2005). Much of this seems to rely on anti-inflammatory cytokines such as TGF-β and IL-10 either directly from dying cells or following uptake by neighboring phagocytes with the assumption that these cytokines are potent inhibitors of inflammatory processes such as leukocyte infiltration and macrophage/neutrophil function. This concept is largely an extrapolation from studies on ultraviolet (UV) radiation or low dose ionizing radiation. Nonetheless, there is growing evidence from clinical and pre-clinical studies that would support this therapeutic radon concept of rebalancing inflammatory/immune networks.
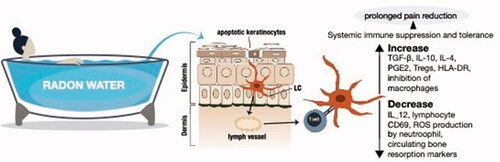
Figure 5. Alpha therapy is an effective treatment for bone metastases. Radionuclides such as radium which home to the bone inherently execute targeted therapy of bone metastases.
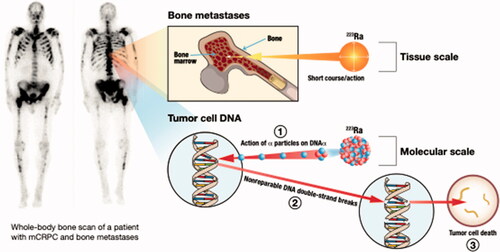