Figures & data
Figure 1. Relationship between Notch modular structure and its activation mechanism. (A) The modular structure of Drosophila Notch and its ligands Serrate and Delta. Vertebrate Notch and ligand homologues have similar domain organisation with some minor differences in number of EGF-like modules in some cases. The seven ankyrin (Ank) domain repeats in the intracellular domain are a highly conserved feature. The heterodimer region (HD) contains a Furin cleavage site known as S1 and processing of Notch during its secretory trafficking results in a heterodimeric molecule expressed at the cell surface, held together through non-covalent interactions in the HD region. S1 processing may not be significant for Drosophila Notch however (Kidd & Lieber, Citation2002). Locations of sequenced Drosophila classical Notch alleles are indicated. Arrows indicate missense mutations, * indicates frame shift or stop codon. Red arrows and red * indicate loss of function, blue arrows and blue * indicate gain of function. Green arrow indicates mutant with complex interpretation. Orange labelled mutants are recessive lethal, others are homozygous viable with variety of adult phenotypes. TS1 is a temperature sensitive allele. Further details of Notch allele phenotypes can be obtained from FlyBase (http://flybase.org). (B) Ligand-induced Notch activation mechanism. Notch is activated by binding of membrane bound ligands expressed on adjacent cells through the EG8-12 region of the receptor and the C2 to EGF3 region of the ligands. Endocytosis of the ligand applies tension on Notch extracellular domain, which causes a local unwinding of the Negative Regulatory Region (NRR) structure. Engagement of Notch with the endocytic machinery of the signal receiving cell may also contribute to this mechano-transduction mechanism. This structural change exposes a proteolytic cleavage site (S2), which lies in the HD region, for processing by Adam10/Kuzbanian metalloprotease. After removal of the bulk of the extracellular domain, the remaining membrane tethered Notch intracellular domain (NICD) is released by γ-secretase processing and NICD is transported to the nucleus through the action of two nuclear localisation sequences (nls), which flank the Ank-domain region. The PEST region (Proline Serine Glutamate Threonine rich) regulates NICD turnover and limits the signal activation. C) Crystal structure of the NRR and HD region from human Notch1 (Gordon et al., Citation2009). LNR (Lin12 Notch repeats) and HD region indicated by colour coded labels. Blue side chain V1721 indicates S2 cleavage site. In the inactive confirmation this site is protected in the core of the structure through interactions with hydrophobic side chains L1481 and F1483 indicated in red. Grey spheres indicate location of Calcium ions. D) Crystal structure (Choi et al., 2009) of human Notch1 Ram-Ank region of NICD complex (green) with the CSL transcriptional activator (yellow) and recruited coactivator protein Mastermind (Mam, pink), bound together as a complex to a DNA helix (Refer online version for colour figure).
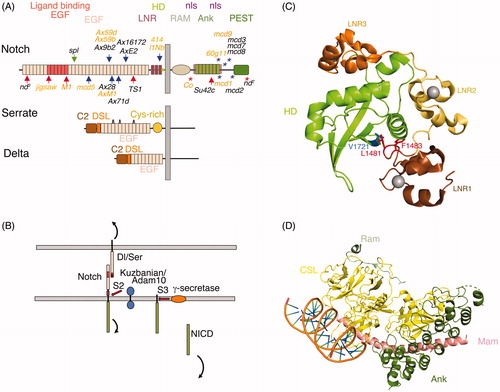
Figure 2. The structure of the Notch/ligand complex. (A) EGF11-13 from human Notch1 (Cordle et al., Citation2008) forms an extended rigid structure with EGF-EGF module interfaces stabilised by Calcium (grey sphere) binding sites (purple side chains) and hydrophobic packing interactions (green side chains). Side chains L468, I477 labelled in blue indicate residues whose mutation reduces Notch/ligand binding and signalling (Whiteman et al., Citation2013). (B) Crystal structure of human Jag-1 C2 to EGF3 (Chillakuri et al., Citation2013). Magenta labelled side chain indicates a conserved F207 residue whose mutation strongly affects binding and Notch signal activation when altered in Drosophila Serrate. Yellow side chains F199 and R203 indicate conserved positions, which when mutated in Drosophila Serrate strongly reduce signalling but have weak affects on binding. They further can act in a dominant negative manner when expressed in vivo in Drosophila wing discs (Cordle et al., Citation2008). (C) A crystal structure of a complex of rat Notch1 EGF8-12 and Jag1 C2-EGF3. Arrow shows approximate position of hinge bending conformational change compared to Jag1 crystallised alone. This conformational change is proposed to facilitate catch bond behaviour (Luca et al., Citation2017). (D) Detail of the EGF11/12 interface with ligand C2/DSL region. Side chains highlighted in (A) and (B) are coloured as above. Additional side chains highlighted are labelled. Jag1 F207 is deeply buried in a hydrophobic pocket supplied by Notch EGF11 comprising P422 and F436 (dark blue) that are a conserved part of the general EGF-module consensus sequence together with the R448 side chain (cyan), which is conserved within EGF11. The Jag1 F199 and R203 engage with conserved EGF11 residues E423 and H424 (Red). The former forming a salt bridge with Arg203 and also a hydrogen bond with conserved DSL domain Y210. In EGF12 side chains L468 and I477 (blue) form hydrophobic interactions surface with F94 and F126 in the C2 domain (side chains shown in orange). O-fucose attached to conserved threonine in EGF 12 (black) also contributes to this interface. (E) Detail of Notch EGF8-10 interface with Jag1 EGF1-3. Hydrophobic interactions mediated by conserved residues from Notch (dark blue) and from Jagged EGF1-3 (green). Black side chain represents an O-fucosylated T311, which contributes to binding affinity, making a H-bond with N298 in EGF3 of Jag-1. In purple is V324, which is the location of the Drosophila Njigsaw allele, whose mutation affects Serrate signalling but not activation through Delta (Yamamoto et al., Citation2012) (Refer online version for colour figure).
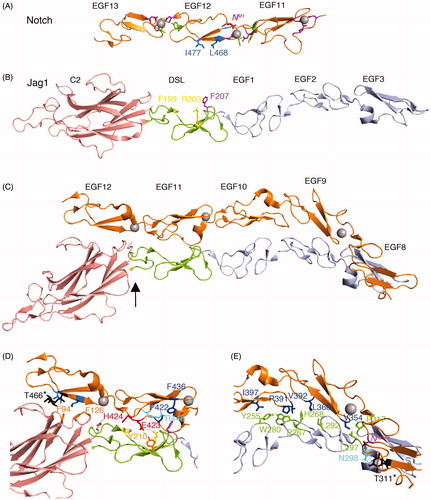
Figure 3. The Notch Dll4 complex. Crystal structure of the Dll4 C2 to EGF1 region in complex with Notch1 EGF11-13 (Luca et al., Citation2015). The C2 domain makes similar contacts with EGF12 of Notch1 to those observed for Jag1 ligand, involving conserved Phenylalanine residues (F76, F109). The DSL domain residue F195 (conserved with Jag1 F207) is similarly buried in the EGF11/Jag1 interface and Leu187, a conserved substitution for Jag1 F199, is similarly involved. Differences in detail can be discerned between the Jag1 and Dll4 complexes. The EGF11 conserved R448 forms a salt bridge with DLL4 D218 (orange) and its side chain is buried by the DSL domain residue Y216 (grey), side chain interactions which do not exist for the Jag-1 complex.
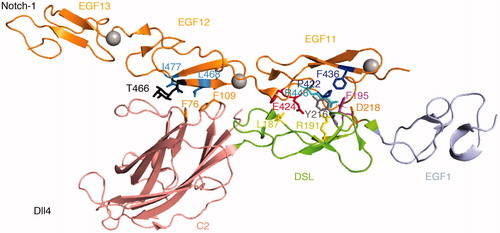
Figure 4. DSL-ligand-independent activation of Notch in the endo/lysosomal pathway. Notch can be endocytosed through Clathrin-dependent and independent routes (Shimizu et al., Citation2014). Clathrin-mediated endocytosis is promoted through interaction with the E3 ubiquitin ligase protein Deltex (Dx). The latter also acts to promote Notch retention on the endosomal membrane rather than being transferred into intraluminal vesicles of the maturing endosome. On lysosome fusion, mediated by Rab7 and the HOPS complex, the extracellular domain of Notch is removed, independently of the activity of Adam10, and NICD is released by γ-secretase activity. Suppressor of deltex (Su(dx)) also interacts with NICD and promotes Notch endocytosis through a lipid raft-dependent and Clathrin-independent route (Shimizu et al., Citation2014). Su(dx) also acts via Notch ubiquitination to promote Notch internalisation into intraluminal vesicles where it is sequestered from activation and is degraded on lysosomal fusion. If the ubiquitin ligase of Su(dx) is inactive then Notch is retained on the endosomal membrane and can be activated in this membrane domain by an Adam10-dependent activity (Shimizu et al., Citation2014). Additional interactions at the cell surface through Crumbs and ZO-1 regulate these alternative mechanisms of activation by limiting Notch Dx-dependent endocytic trafficking of Notch (Nemetschke & Knust, Citation2016; Shimizu et al., Citation2017).
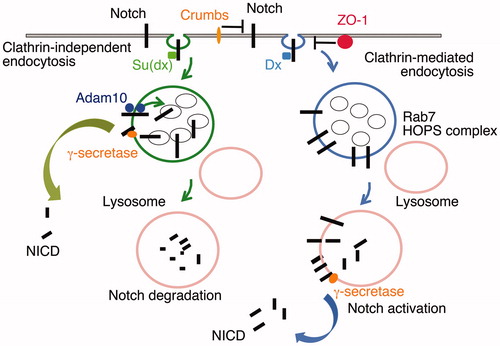