Figures & data
Figure 1. cDNAs encoding small ankyrin and constructs used in these experiments. (A) Small ankyrin consists of an N-terminal sequence of 29 amino acids that is highly hydrophobic, and a hydrophilic sequence of 126 amino acids, the last 15 of which were used to generate the p6 antibody. (B,C) Constructs encoding the lacZ gene product, β-galactosidase, with a C-terminal myc epitope tag, with (B) and without (C) the N-terminal 29 amino acid hydrophobic sequence of sAnk1 at the N-terminus. (D,E) Constructs encoding DS-Red, with (D) and without (E) the N-terminal 29 amino acid hydrophobic sequence of sAnk1 at the N-terminus. A key to the different sequences examined is given below (E) Not drawn to scale.
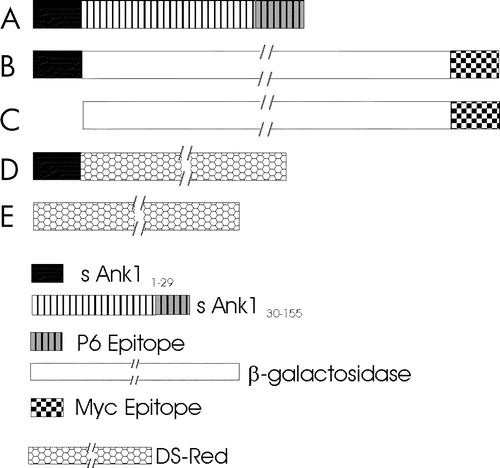
Figure 2. Extraction of proteins carrying the N-terminal hydrophobic sequence of sAnk1 from isolated SR vesicles. Isolated SR vesicles were incubated at RT in solutions of buffered saline (PBS), 6 M urea, 50 mM ethanolamine, pH 11, and buffered saline containing 2% Triton X-100 (Tx). After incubation, samples were subjected to centrifugation, and the distribution of sAnk1 between the supernatant and pellet fractions was examined by SDS-PAGE and immunoblotting with anti-p6 antibody. Parallel lanes were assayed for SERCA by SDS-PAGE and staining with Coomassie brilliant blue. sAnk1 and SERCA behaved similarly, as each were solubilized to significant extents by the detergent solution but not by other treatments.
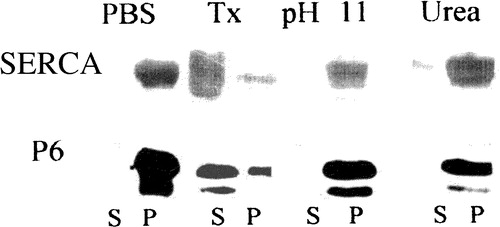
Figure 3. sAnk1 partitions into Triton X-114. SR vesicles were extracted with a warm solution of Triton X-114, and the extracted materials were then cooled to induce phase separation. The aqueous and detergent phases were assayed for their content of sAnk1 by SDS-PAGE and immunoblotting, or for their content of SERCA by SDS-PAGE and staining with Coomassie brilliant blue. Both proteins partition into the detergent phase, consistent with their identification as integral membrane proteins. Bovine serum albumin remains in the aqueous phase.
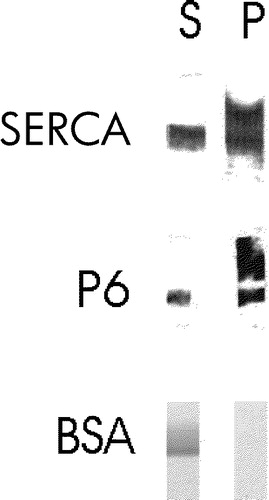
Figure 4. Treatment of isolated SR vesicles with low concentrations of trypsin removes the p6 epitope. Isolated SR vesicles were treated for 10 min at RT with 0, 5, 10, 20, 50 and 100 ng ml−1 of trypsin in PBS, boiled in SDS-PAGE sample buffer and subjected to SDS-PAGE. Calsequestrin (CS) and the C-terminal region of sAnk1 containing the p6 epitope were labeled by immunoblotting; SERCA was visualized with Coomassie brilliant blue. Concentrations of trypsin above 5 ng ml−1 removed the p6 epitope from sAnk1 but had no effect on SERCA or calsequestrin, consistent with the presence of the C-terminus of sAnk1 on the cytoplasmic surface of the SR.

Figure 5. Oligomers of sAnk1 are present under non-reducing conditions. Fresh homogenates of rat hindlimb muscle were mixed with 0.7 M 2-mercaptoethanol in SDS-PAGE sample buffer supplemented with 4 mM TCEP, or SDS-PAGE sample buffer lacking all reducing agents. Each sample was then either boiled for 5 min, or incubated for 15 min in sample buffer at 37°C. After electrophoresis, samples were transferred to nitrocellulose paper and immunoblotted with anti-p6 antibodies. All reduced samples showed a major band at ∼21 kDa, with smaller amounts of label apparent at ∼38, 58, 75 and 100 kDa. All unreduced samples failed to show significant amounts of label at 21 kDa and instead showed major bands at ∼46 and ∼80 kDa, consistent with the presence of dimers and tetramers of sAnk1.
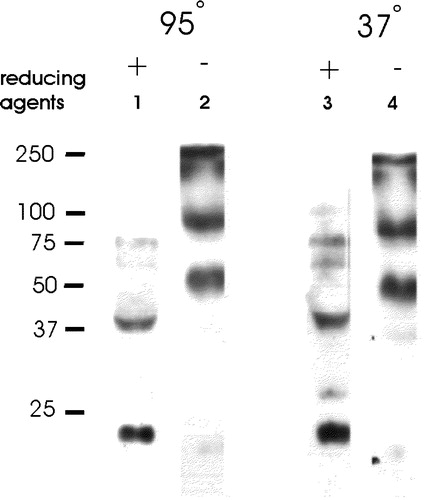
Figure 6. The hydrophobic N-terminal sequence of sAnk1 targets a reporter protein to intracellular membranes in HEK 293 and COS-7 cells. The plasmid cDNAs encoding either myc-tagged β-galactosidase (A,C) or DS-Red (B,D), with (A,B) and without (C,D) the N-terminal hydrophic sequence of sAnk1 (see ), were introduced into HEK 293 (A,C) or COS-7 (B,D) cells. One day later, transfected COS-7 cultures were fixed and observed under fluorescence optics; HEK 293 cultures were fixed, permeabilized, labeled by immunofluorescence with antibodies to the myc tag or to β-galactosidase, and observed. The forms of DS-Red and lac Z containing the N-terminal hydrophobic sequence of sAnk1 were present in reticular or vesicular compartments within the cells (A,C), whereas the forms lacking this sequence were present more uniformly in the cytoplasm (B,D). Scale bar, 5 µm.

Figure 7. Membrane association in mononucleate cells of lacZ and DS-Red constructs with and without the N-terminal hydrophobic sequence of sAnk1. (A,B) COS-7 cells (A) or HEK 293 cells (B) were transfected with plasmid cDNAs encoding DS-Red (A) or lac Z that carried a C-terminal myc epitope tag (B), each with the hydrophobic sequence of sAnk1 at the N-terminus (see ). COS-7 cells were also transfected with plasmid cDNA encoding DS-Red, without the N-terminal sequence of sAnk1 (C). One day after transfection, cells were homogenized and subjected to brief centrifugation. The pellets were washed with buffered saline and, after a brief centrifugation, the distribution of the reporter proteins was examined in the pellet and supernatant fractions. The DS-Red construct introduced into COS-7 cells carrying the N-terminal hydrophobic sequence of sAnk1 was enriched in the pellet and absent from the supernatant fractions (A, PBS). The construct lacking this sequence was only present in significant amounts in the supernatant fraction (C, PBS). The lac Z construct introduced into HEK 293 cells carrying the N-terminal hydrophobic sequence of sAnk1 behaved similarly to the DS-Red construct with the sAnk1 sequence, shown in A (panel B). The crude membrane fractions containing DS-Red or lac Z carrying the N-terminal hydrophobic sequence of sAnk1 were also extracted with solutions containing 2% Triton X-100 or 6 M urea, or buffered at pH 11. Only Triton X-100 solubilized the reporter proteins (A,B: Tx). These results suggest that the N-terminal hydrophobic sequence of sAnk1 is sufficient to confer DS-Red and lac Z with the solubility properties of integral membrane proteins, similar to sAnk1 itself.
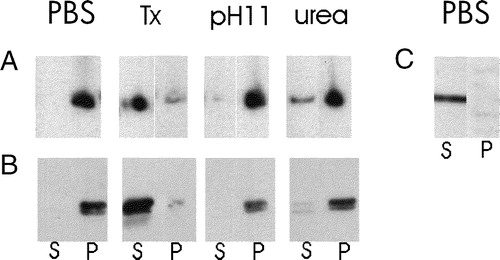
Figure 8. The hydrophobic N-terminal sequence of sAnk1 targets a reporter protein to the network SR of skeletal muscle. The plasmid cDNAs encoding myc-tagged β-galactosidase with (A,C,G,J: 1–29) and without (E: con) the N-terminal hydrophobic sequence of sAnk1 (see ), were injected into muscle to transfect muscle fibers. Seven to 10 days later, muscle was perfusion fixed, collected, snap frozen and immunolabeled for the transgene product with antibodies to the myc epitope or to β-galactosidase (A,C,E,G,I), as well as for endogenous sAnk1, recognized with the anti-p6 antibody (B,D,F,H,J: P6). (A–F) Cross-sections of transfected skeletal muscle fibers. Lac Z carrying the N-terminal hydrophobic sequence of sAnk1 (A,C, arrows) codistributed with the endogenous sAnk1 in the SR (B,D, arrows). Lac Z lacking this sequence (E) did not target to the SR (F). (G–J) Longitudinal sections of muscle showing transfected skeletal muscle fibers were labeled as in A–F. The protein encoded by the transgene concentrates in structures that surround the M lines (white arrows) and Z disks (grey arrows) of the myofibers (G,I), which also contain sAnk1 (H,J). Whereas sAnk1 concentrates around Z disks with lower amounts around M lines (Zhou et al. [Citation1997]), the reporter protein appears to concentrate around M lines with lower amounts around Z disks. Scale bars, 5 µm.
![Figure 8. The hydrophobic N-terminal sequence of sAnk1 targets a reporter protein to the network SR of skeletal muscle. The plasmid cDNAs encoding myc-tagged β-galactosidase with (A,C,G,J: 1–29) and without (E: con) the N-terminal hydrophobic sequence of sAnk1 (see Figure 1), were injected into muscle to transfect muscle fibers. Seven to 10 days later, muscle was perfusion fixed, collected, snap frozen and immunolabeled for the transgene product with antibodies to the myc epitope or to β-galactosidase (A,C,E,G,I), as well as for endogenous sAnk1, recognized with the anti-p6 antibody (B,D,F,H,J: P6). (A–F) Cross-sections of transfected skeletal muscle fibers. Lac Z carrying the N-terminal hydrophobic sequence of sAnk1 (A,C, arrows) codistributed with the endogenous sAnk1 in the SR (B,D, arrows). Lac Z lacking this sequence (E) did not target to the SR (F). (G–J) Longitudinal sections of muscle showing transfected skeletal muscle fibers were labeled as in A–F. The protein encoded by the transgene concentrates in structures that surround the M lines (white arrows) and Z disks (grey arrows) of the myofibers (G,I), which also contain sAnk1 (H,J). Whereas sAnk1 concentrates around Z disks with lower amounts around M lines (Zhou et al. [Citation1997]), the reporter protein appears to concentrate around M lines with lower amounts around Z disks. Scale bars, 5 µm.](/cms/asset/87066d36-ca5c-4f52-a8fd-e65b9fef3be6/imbc_a_124409_f0008_b.jpg)
Figure 9. Model of sAnk1 in the network SR membrane, and its relationship to its ligands, obscurin and titin. sAnk1 is anchored to the network SR membrane by its N-terminal hydrophobic sequence. Molecular modeling predicts that, following removal of its N-terminal methionine residue, only 2 amino acids are exposed on the lumenal surface of the SR membrane. The entire sequence C-terminal to the transmembrane domain is exposed to the cytoplasm, where it can interact with obscurin and titin at the level of the Z disks, or obscurin alone at the level of the M lines. sAnk1 is pictured as disulfide-linked dimers, although tetramers, and even larger oligomers, may also be present. These dimers may bind to two molecules of obscurin simultaneously at either Z disks or M lines, with one molecule of obscurin and one molecule of titin at Z lines, or with two molecules of titin at Z-lines. The titins that are anchored at the Z lines of the sarcomere pictured here, but that extend towards the M lines of nearby sarcomeres, are not drawn as complete molecules. Not drawn to scale. This figure is reproduced in color in Molecular Membrane Biology online.
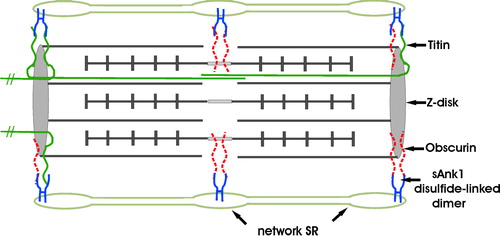