Figures & data
Figure 1. Expression and purification of Atm1p. (A) A fusion protein construct (OmpA-His8-ATM1-Strep) was used for heterologous synthesis of Atm1p in E. coli. The fusion protein consisted of an OmpA signal sequence, an eight codon long N-terminal His-tag, the ATM1 gene lacking the first 26 codons, and a C-terminal Strep-tag II. The corresponding DNA construct was cloned into the vector pASK-IBA1 (IBA Göttingen). The E. coli derived OmpA signal sequence facilitates targeting and insertion of the protein into the bacterial inner membrane. (B) E. coli BL21 cells carrying the plasmid described in A were grown in the presence or absence of anhydrotetracycline (Tet) to induce gene expression of the ATM1 fusion gene. Cell extracts were analysed by immunostaining for Atm1p or the Strep-tag using specific antibodies. A similar analysis was performed for synthesis of an Atm1p fusion protein lacking the bacterial OmpA signal sequence. C) Atm1p purification from E. coli BL21 after induction of gene expression by anhydrotetracycline. E. coli inner membranes were isolated and solubilized with the detergent n-dodecylmaltoside. The protein was purified on a StrepTactin Macroprep column. Fractions of each isolation step were subjected to SDS-PAGE, stained with Coomassie Brilliant Blue (lower panel), or electroblotted and immunodecorated with an Atm1p-specific antibody (upper panel). Lanes 1 and 2: Whole cell extracts without and with induction by anhydrotetracycline (50 µg protein); lane 3: solubilized membranes of induced E. coli (50 µg protein); lane 4: column flow-through (50 µg); lane 5: wash fraction; lane 6: eluted, purified protein (4.1 µg). (D) Purified Atm1p was applied to a gelfiltration column (Shodex Protein KW-804 column) and two peaks with molecular masses of 58 and 260 kDa were eluted.
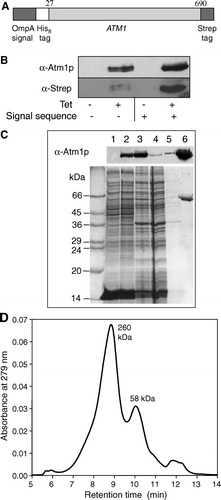
Figure 2. Purified, solubilized Atm1p has an ATPase activity that is stimulated by lipids. (A) Purified Atm1p was incubated at 20°C and 30°C in 100 mM Tris-HCl pH 8.0 containing 0.025% n-dodecylmaltoside and 1 mM ATP. ATPase activity was followed by phosphate release using a colorimetric assay (Lill et al. [Citation1989]). (B) The four most abundant phospholipids of the mitochondrial inner membrane and purified Atm1p (in 0.025% n-dodecylmaltoside solution) were mixed and incubated at 30°C for 30 min. Aliquots containing 0.14 µg Atm1p and 7.4 µg lipid were tested for their ATPase activity. Composition of lipid mixtures: PC/PE/PI: 45% DOPC, 37% DOPE, 18% PI; CL/PC/PE/PI 18% cardiolipin, 41% DOPC, 39% DOPE, 2% PI. Bars represent the standard errors of three independent experiments.
![Figure 2. Purified, solubilized Atm1p has an ATPase activity that is stimulated by lipids. (A) Purified Atm1p was incubated at 20°C and 30°C in 100 mM Tris-HCl pH 8.0 containing 0.025% n-dodecylmaltoside and 1 mM ATP. ATPase activity was followed by phosphate release using a colorimetric assay (Lill et al. [Citation1989]). (B) The four most abundant phospholipids of the mitochondrial inner membrane and purified Atm1p (in 0.025% n-dodecylmaltoside solution) were mixed and incubated at 30°C for 30 min. Aliquots containing 0.14 µg Atm1p and 7.4 µg lipid were tested for their ATPase activity. Composition of lipid mixtures: PC/PE/PI: 45% DOPC, 37% DOPE, 18% PI; CL/PC/PE/PI 18% cardiolipin, 41% DOPC, 39% DOPE, 2% PI. Bars represent the standard errors of three independent experiments.](/cms/asset/380c9b3b-d162-4b4a-984e-71dafc70f3b7/imbc_a_147346_f0002_b.gif)
Figure 3. Reconstitution of Atm1p into proteoliposomes. (A) The reconstitution of purified Atm1p was carried out by detergent removal as described in Materials and Methods. After each reconstitution step an aliquot (A1–A5) of the suspension was removed and tested for ATPase activity and protein content. The aliquots were chosen as follows: A1, mixture of lipid and protein; A2, same after 1 h incubation at room temperature; A3 and A4, samples after first and second detergent removal steps; A5, floated sample obtained after flotation centrifugation (see Materials and Methods). A control sample (Con) containing only purified, solubilized Atm1p and no lipids was mock-treated in 0.025% n-dodecylmaltoside-containing buffer at the same temperatures as the reconstitution samples. Specific activities of the Atm1p ATPase are given for four independent experiments (error bars represent the standard deviation). (B) Membrane orientation of reconstituted Atm1p. Floated proteoliposomes (equivalent to sample A5) were treated with proteinase K (PK) and Triton X-100 (Tx-100) as indicated. Atm1p was analysed by silver staining or detected by the Strep-tag (α-Strep-tag) affinity detection system.
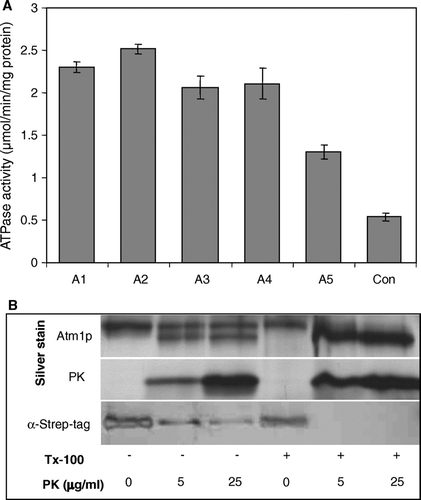
Figure 4. Characterization of the ATPase activity of reconstituted Atm1p. (A) The ATPase activity of Atm1p in proteoliposomes (corresponding to sample A4 in A) is inhibited by vanadate. Reconstituted Atm1p was incubated at 30°C in 100 mM Tris pH 8.0, 50 mM NaCl, 2 mM MgCl2, 1 mM ATP in the presence of the sodium vanadate concentrations as indicated. The ATPase activity was estimated as in . (B) The Km- and Vmax-values for ATP of reconstituted Atm1p in proteoliposomes were calculated as 0.13 mM (± 0.03) and 1.9 (± 0.4) nmol phosphate/min/µg, respectively using WordPadPrism™ non-linear regression analysis. For each ATP concentration chosen the initial rate of the reaction was determined by measurement of the ATPase activity at five time points. The data are presented according to Lineweaver-Burk.
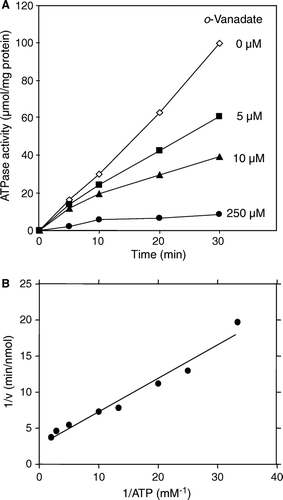
Figure 5. Stimulation of the Atm1p ATPase activity by thiol compounds. (A) Atm1p-containing proteoliposomes were floated on a sucrose gradient and tested for their ATPase activity in the presence of different thiol-containing compounds (1 mM final concentration). The figure shows the mean of three independent determinations, relative to the value obtained without further addition. GSH, reduced glutathione; GSSG, oxidized glutathione; DTT ox, oxidized DTT. (B) The ATPase activity of floated Atm1p proteoliposomes was measured in the presence of increasing concentrations of cysteine, DTT, GSH, and of the reducing agents Tris(2-carboxyethyl)phosphine (TCEP) and Tris(2-cyanoethyl)phosphine (TCP). For the latter two reagents, no stimulatory effect was seen, whereas the thiol-containing compounds increased the ATPase activity in a concentration-dependent fashion.
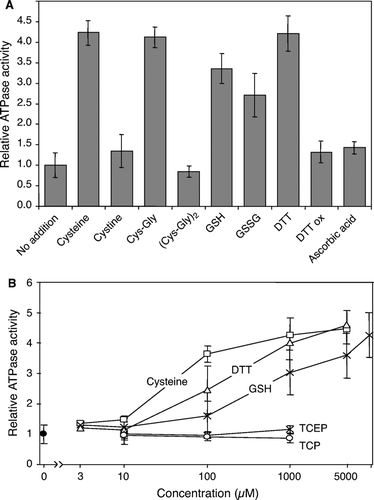
Figure 6. Efficient stimulation of Atm1p ATPase activity by cysteine-containing peptides or proteins. Atm1p was reconstituted into proteoliposomes, floated on a sucrose gradient and tested for ATPase activity in presence of different concentrations of peptides (A and B) and proteins (C). Part A shows two cysteine-containing peptides (P1-C1, P2-C1) and two peptides without a cysteine residue (P1-S1, P3-C0). Part B documents the efficient stimulation of the Atm1p-ATPase activity by a peptide containing four cysteine residues (P4-C4), but not by its derivative, where the cysteine residues were replaced by serine residues (P4-S4). In part C the apo- and holoforms of the S. elongatus ferredoxin PetF were incubated with Atm1p-containing proteoliposomes and the ATPase activity was recorded as above. Note that the preparation contains about 15% apoform. For comparison the ATPase activity of the proteoliposomes was recorded in the presence of cysteine. All data are presented relative to the value obtained without further additions. Data are the mean of three independent experiments; the bars represent the standard deviation.
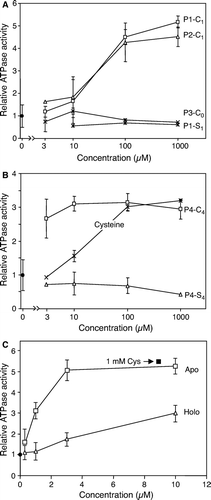