Figures & data
Figure 1. Membrane fusion induced by peptide145–164 depends on the negative charges of its acidic residues. (A) Effect of the modification of the acidic residues with DCCD. Equal amounts of unlabeled vesicles and vesicles labeled with Rh-PE and NBD-PE were incubated with the peptide145–164 or the peptide pre-incubated with DCCD. The vesicles were composed of PC:PS (1:3) and were prepared in 20 mM MES, 30 mM Tris buffer, pH 6.0, in a final phospholipid concentration of 0.1 mM. Peptide-induced membrane fusion activity was measured by the increase in the NBD-PE fluorescence. NBD-PE was excited at 470 nm, and the intensity was collected at 530 nm, during 10 min. The final peptide concentration was 20 µg/ml. (B) Effect of pH. Fusion reaction was measured as described above except that the pH was adjusted to 7.5, 6.0 or 4.0, as indicated in the Figure.
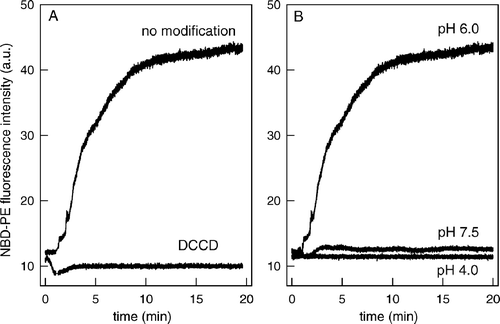
Figure 2. Labeling of the peptide145–164 with DCCD. ESI-MS spectra were recorded to monitor the addition of DCCD groups to the Asp and Glu residues of the peptide145–164. The peptide (20 µg/ml) was incubated for 1 h with DCCD in 20 mM MES, 30 mM Tris buffer, pH 6.0. Before analysis, the buffer was removed on ZipTip C18 and the peptide was solubilized in 50% acetonitrile/ 1% formic acid (v/v). The different populations observed (arrows) correspond to the addition of 1, 2 and 3 DCCD molecules, respectively. The accuracy of mass measurement was in the order of 8 ppm.
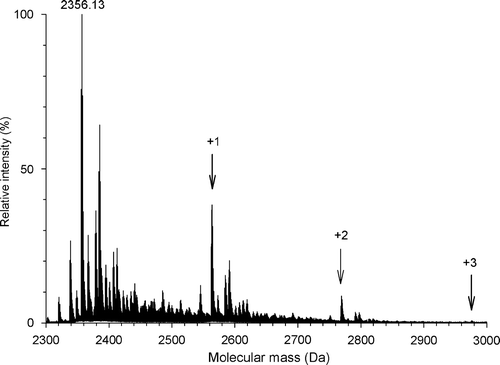
Figure 3. Acidic residues are involved in the formation of a hydrophobic region. (A) ANS binding as a function of pH. Peptide145–164 was diluted to a final concentration of 20 µg/ml in 20 mM MES, 30 mM Tris buffer, pH as indicated in the Figure, and incubated with 1 µM ANS. ANS was excited at 360 nm and the emission was collected at 492 nm. (B) Peptide145–164 without modification (—) or modified with DCCD (…) were incubated with 1 µM ANS in 20 mM MES, 30 mM Tris buffer, pH 6.0, and the ANS fluorescence spectra were collected after excitation at 360 nm.
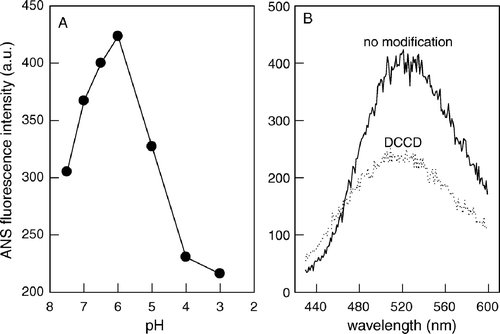
Figure 4. His148, Asp153 and Glu158 are important for peptide hydrophobicity and fusion. (A) Amino acid sequences of the peptides used in this study. Peptide wt used in this study corresponds to VSV G protein residues between 145 and 164. Six different mutants were also synthesized: His148, His149, Glu154 or Glu158 were substituted by Gln residues, and Asp153 or Asp161 were substituted by Asn residues. The dots represent wt residues. (B) Peptide145–164 or the mutant peptides were incubated with 1 µM ANS in 20 mM MES, 30 mM Tris buffer, pH 6.0, and the ANS fluorescence spectra were collected after excitation at 360 nm. (C) Kinetics of membrane fusion by peptide145–164 or mutant peptides. Equal amounts of unlabeled vesicles and vesicles labeled with Rh-PE and NBD-PE were incubated with each of the peptides and the membrane fusion was measured monitoring the increase in NBD-PE fluorescence. The vesicles were composed of PC:PS (1:3) and were prepared in 20 mM MES, 30 mM Tris buffer, pH 6.0, in a final phospholipid concentration of 0.1 mM. NBD-PE was excited at 470 nm, and fluorescence intensity was collected at 530 nm during 10 min.
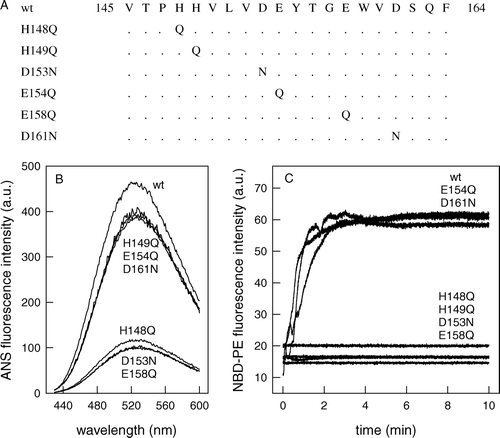
Figure 5. Peptide oligomerization is required for hydrophobicity and fusion. (A) Kinetics of membrane fusion at different peptide concentration. Equal amounts of unlabeled vesicles and vesicles labeled with Rh-PE and NBD-PE were incubated with the peptide145–164 in a final concentration of 3, 6, 12, 18, 30 and 100 µg/ml, and the membrane fusion was measured monitoring the increase in NBD-PE fluorescence. The vesicles were composed of PC:PS (1:3) and were prepared in 20 mM MES, 30 mM Tris buffer, pH 6.0, in a final phospholipid concentration of 0.1 mM. NBD-PE was excited at 470 nm, and the fluorescence intensity was collected at 530 nm during 10 min. (B) Percentage of fusion after 10 min as a function of peptide concentration. (C) ANS binding as a function of peptide concentration. Peptide145–164 (•) and the mutants H148Q (○), H149Q (▪), D153N (▾), E154Q (▵), E158Q (▾), and D161N (▿) were diluted in 20 mM MES, 30 mM Tris buffer, pH 6.0, to a final concentration as indicated in the Figure, and incubated with 1 µM ANS. ANS was excited at 360 nm and the emission was collected at 492 nm.
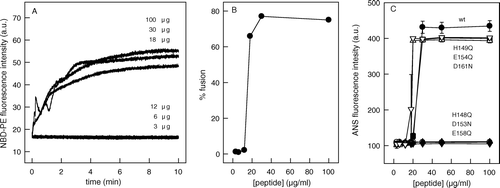
Figure 6. DCCD labeling is dependent on peptide concentration. Peptide145–164 diluted to a final concentration of 3 µg/ml (A) or 100 µg/ml (B) was modified for 1 h with DCCD at pH 6.0 and ESI-MS spectra were recorded. No labeling was observed with 3 µg/ml and a labeling comparable to the one described in was observed for 100 µg/ml.
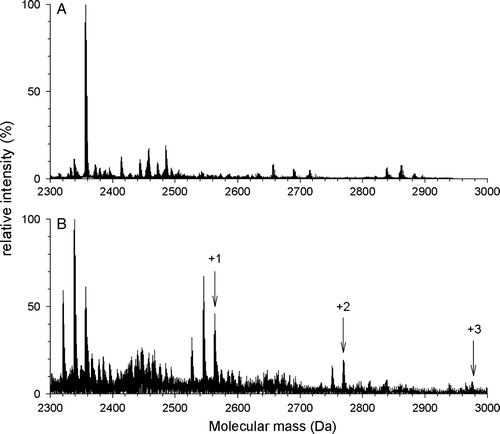
Figure 7. Secondary structure measurements. (A) ATR-FTIR spectra were recorded from thin films obtained by slowly evaporating a sample containing 10 µg of peptide145–164 (at pH 7.5 or 6.0, as indicated in the Figure) on an attenuated total reflection element. The samples were rehydrated by flushing D2O-saturated N2 for 30 min at room temperature. (B) The wild-type spectrum was compared with the spectra obtained, at pH 6.0, for the mutants H148Q, H149Q, D153N and E158Q, as indicated in the Figure.
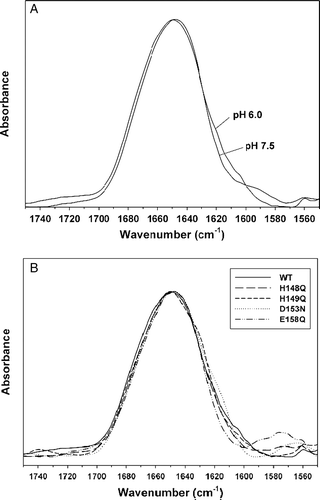
Figure 8. Kyte-Doolittle plots of fusion peptide hydropathy. Hydrophobic profile of VSV peptide[145–164] and HIV-1 fusion peptide (sequence VGIGALFLGFLGAAGSTHGA). The hydropathy of these peptides was plotted from the amino terminus to the carboxy terminus by averaging hydropathy values over a window of 5 residues. More positive values are assigned to more hydrophobic residues.
![Figure 8. Kyte-Doolittle plots of fusion peptide hydropathy. Hydrophobic profile of VSV peptide[145–164] and HIV-1 fusion peptide (sequence VGIGALFLGFLGAAGSTHGA). The hydropathy of these peptides was plotted from the amino terminus to the carboxy terminus by averaging hydropathy values over a window of 5 residues. More positive values are assigned to more hydrophobic residues.](/cms/asset/706f777c-69c6-46dd-9c9d-55e1bfeadeea/imbc_a_178041_f0008_b.gif)