Figures & data
Figure 1. In vitro methods for translocation assessment, using FRET and quenching methodologies. Peptide molecules are represented by empty rectangles and additional non-translocating compounds by circles; peptide molecules that have interacted with those compounds are dashed; phospholipids labelled with an acceptor for the peptide's fluorescence are indicated by light grey headgroups. (A) Measurement of the inaccessibility of the peptide to a non-translocating entity with the ability to digest, sequester or permanently quench it; the interaction between the two prevents the peptide from acting as a FRET donor to the labelled phospholipids; small FRET reductions will indicate that the peptide was protected from that interaction; this indicates that the peptide is buried in the membrane or is located in lumen. (B) Detection of inward peptide movement; after the initial adsorption interaction of the peptide with asymmetrically acceptor-labelled vesicles (despite the representation, both inner-leaflet and outer-leaflet labelling can be used), translocation or membrane penetration processes causes the mean distance between peptide and labelled phospholipids to increase (assuming an outer-leaflet labelling) and a concomitant reduction of FRET. If the peptide has quenching capabilities, a quenchable probe can be used to symmetrically label the vesicles; translocation can then be identified by full probe quenching, which is only possible if the peptide reaches the inner bilayer surface. (C) Detection of peptide in the inner phase; in the case of translocation, digestion by encapsulated trypsin (solid circles) reduces the overall amount of peptide available for membrane interaction and, consequently, FRET between peptide and labelled-membrane; trypsin inhibition (dashed circles) is required in the outer phase, prior to peptide addition. (D) Detection of encapsulated peptide escape; in the event of translocation, the peptide fluorescence decreases as it becomes accessible to an externally added, non-translocating quencher; the quencher can, alternatively, be co-encapsulated with the peptide, and the quenching reduction monitored instead. The figure is reproduced in colour in Molecular Membrane Biology online.
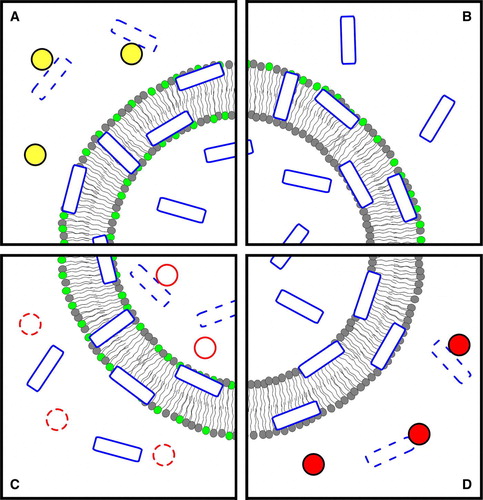
Figure 2. To evaluate the translocation of β-Gal/pep-1 (hexagons/rectangles) complexes across model membranes the following steps were reported Citation[81]: (1) valinomycin was added to K+-loaded vesicles in Na+-buffer to create a negative transmembrane potential; the complex was added to the solution and translocated across bilayer; (2) trypsin (circles) was added to digest non-incorporated complex and free pep-1 or β-Gal (dashed hexagons/rectangles); (3) after digestion, trypsin was inhibited (dashed circles) with phenylmethylsulfonyl fluoride and TX-100 was then added to facilitate the release of internalized β-Gal and allow its quantification (digestion debris were excluded from the last two panels, and inhibited trypsin from the last, to avoid image crowding); (4) β-Gal activity was assayed by MUG (black triangles) hydrolysis to a fluorescent product, 4-MU (lighter triangles). The figure is reproduced in colour in Molecular Membrane Biology online.
![Figure 2. To evaluate the translocation of β-Gal/pep-1 (hexagons/rectangles) complexes across model membranes the following steps were reported Citation[81]: (1) valinomycin was added to K+-loaded vesicles in Na+-buffer to create a negative transmembrane potential; the complex was added to the solution and translocated across bilayer; (2) trypsin (circles) was added to digest non-incorporated complex and free pep-1 or β-Gal (dashed hexagons/rectangles); (3) after digestion, trypsin was inhibited (dashed circles) with phenylmethylsulfonyl fluoride and TX-100 was then added to facilitate the release of internalized β-Gal and allow its quantification (digestion debris were excluded from the last two panels, and inhibited trypsin from the last, to avoid image crowding); (4) β-Gal activity was assayed by MUG (black triangles) hydrolysis to a fluorescent product, 4-MU (lighter triangles). The figure is reproduced in colour in Molecular Membrane Biology online.](/cms/asset/6ae00cf0-ef92-4919-ae05-c4a6d761fbec/imbc_a_210186_f0002_b.jpg)
Table I. Classification of available methods for in vivo and in vitro translocation assessment, and respective advantages and disadvantages.