Figures & data
Figure 1. Rapid actions of corticosteroids in the brain. (A) Rapid feedback inhibition of the stress axis—activated by histamine administration (arrows)—was observed at 2 (but not 10 or 45; stars) min after the onset of corticosterone being infused into anesthetized female rats at a constant rate for 2 h. Delayed inhibition was observed when the rats received a stressor 120 min after corticosterone infusion started. Based on Dallman (Citation2005). (B) The mechanism of rapid inhibitory corticosteroid actions was further explored with electrophysiological methods, recording miniature excitatory postsynaptic currents (mEPSCs; typical recording in inset), each of which reflects the postsynaptic response to a spontaneously released presynaptic glutamate-containing vesicle. Changes in mEPSC frequency usually indicate an altered (presynaptic) release probability of the vesicles, whereas changes in mEPSC amplitude rather point to changes in the expression of postsynaptic receptor subunits. Panels B–D based on Tasker and Joëls (Citation2015). (C) Schematic representation how corticosterone may bind to a membrane-bound version of the glucocorticoid receptor (mbGR) on CRH-producing neurons in the PVN, and then through retrogradely transported endocannabinoids (eCB) reduce the release of glutamate-containing vesicles from presynaptic neurons. The recordings on the right show that the frequency (but not amplitude) of mEPSCs is reduced by the GR-agonist dexamethasone, compared to the control situation prior to dexamethasone. (D) In CA1 pyramidal neurons of the hippocampus, corticosteroids act via mineralocorticoid receptors (mMR) presumably located at (or in the vicinity of) the presynaptic plasma membrane. As shown on the right, this results in an increased frequency—but not amplitude—of mEPSCs during administration of (100 nM of) corticosterone.
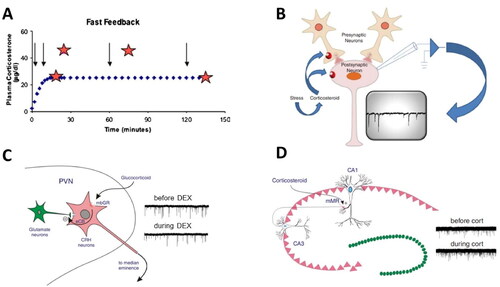
Figure 2. Metaplasticity of corticosterone responses in the basolateral amygdala. (A) Principal neurons in the basolateral amygdala (BLA) respond within minutes to corticosterone with an increase in the mEPSC frequency. A similar increase is also seen when corticosterone is applied for a second time, within 60 min after the first administration. However, with longer delays between the first and second administration, this increase in mEPSC frequency alters into a decrease. Each dot represents the percentual change in mEPSC frequency observed in a particular BLA neuron tested at the delay (after the first administration) indicated on the x-axis. The shift from increased to decreased mEPSC frequency with a delay of >1 h was dubbed “metaplasticity.” (B) Interestingly, some degree of metaplasticity also occurs when corticosterone is administered after the β-agonist isoproterenol, although the effect was less clearly seen and seemed to occur already after a delay of ∼30 min. (C) The metaplasticity observed in relation to isoproterenol and corticosterone may be of relevance for the naturally occurring waves of stress mediators. We tested three conditions, i.e. (i) a situation with only a wave of (a low dose of) isoproterenol (left (i.e. first) wave), reflecting a situation of arousal (top); (ii) a situation with subsequent waves of moderately high doses of isoproterenol and corticosterone (right (i.e. second) wave) respectively, such as may occur during moderate stress (middle); and (iii) a situation with subsequent waves of high doses of isoproterenol and corticosterone, such as may occur during severe stress (bottom). Whereas in the former two situations, BLA neuronal activity is only temporarily high, the latter situation caused a prolonged increase in excitability. All panels are based on Karst and Joëls (Citation2016).
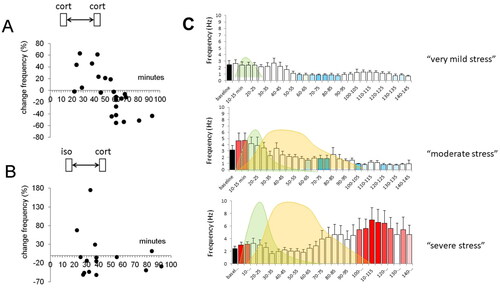
Figure 3. Rapid and delayed effects of stress hormones on cognitive processing. Summary of behavioral effects described in rodents (r) and humans (h) observed shortly after elevation of stress hormones (top left) and with a delay of at least 1 h (top right). The behavioral paradigms that were employed (left) are arranged from (top to bottom) those involving emotional circuits (including the amygdala); via rather routine-based circuits (e.g. involving the striatum); more flexible context-dependent paradigms (involving e.g. the hippocampus); to those related to executive functions like decision-making (involving e.g. the prefrontal cortex). Overall, the studies indicate that directly after stress emotional and routine-based circuits are enhanced in activity, while higher cognitive functions are suppressed. By contract, starting 1 h after stress, the opposite effect is seen. The rapid effects (top-left arrow) most likely involve noradrenergic activation, as well as the effects of neuropeptides like CRH and corticosteroids acting via MR. The delayed effects (arrow on lower right) can be mostly explained by gene-mediated actions via GR.
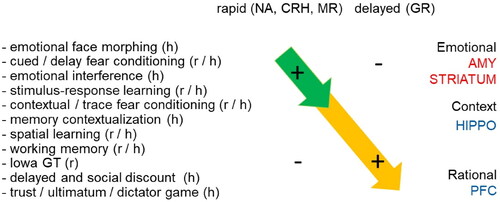