Figures & data
Figure 1. Geometry model and computational domain. (a) AGTB-B1 blade [28] in a cascade [29]. (b) Computational domain with boundary condition.
![Figure 1. Geometry model and computational domain. (a) AGTB-B1 blade [28] in a cascade [29]. (b) Computational domain with boundary condition.](/cms/asset/4cdd0173-4f78-4a61-a3b5-c950a2ad5675/unht_a_1713692_f0001_c.jpg)
Table 1. Cascade geometry and parameters.
Table 2. Boundary conditions and particle properties.
Figure 3. Grid independence study. (a) Mesh view, (b) Film-cooling effectiveness near the blade-leading edge.
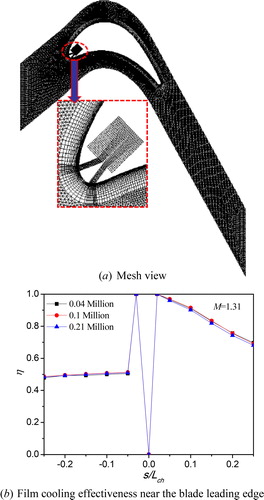
Figure 6. Comparison of film-cooling effectiveness distributions for the cases with various inlet flow angles at four blowing ratios.
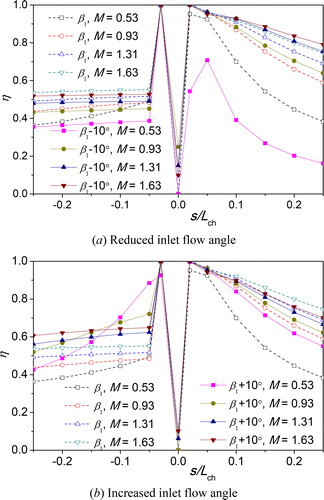
Figure 7. Streamlines and temperature contours near the leading edge for the cases with various inlet flow angles at four blowing ratios.
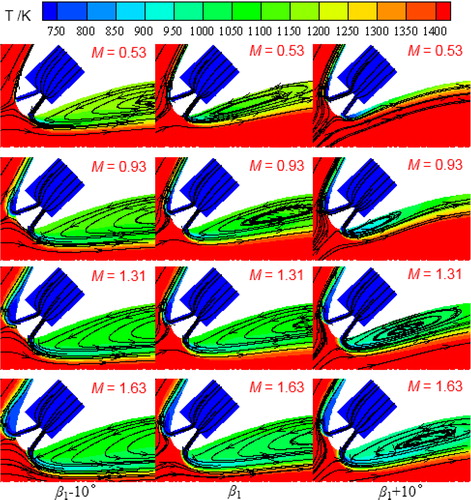
Figure 9. Comparisons of particle trajectories and temperature variations for various particle diameters M= 0.93 and β1=133˚.
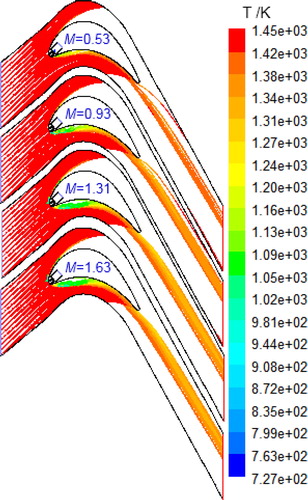
Figure 10. Comparisons of particle trajectories and temperature variations for various inlet flow angles at M=0.53 and 1.63, dp=0.93.
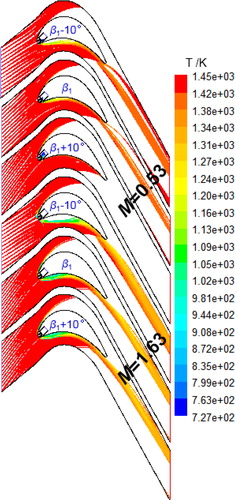
Figure 11. Comparisons of impact and capture efficiencies in different zones based on various particle sizes.
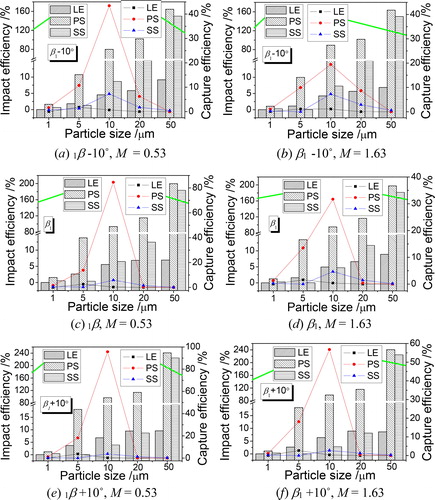