Figures & data
Figure 1. An illustration shows (a) an alternating sinusoidal magnetic field (black) and the corresponding Barkhausen signal (red), and (b) the rectified Barkhausen bursts during (i) ascending magnetic field excitation and (ii) descending magnetic field excitation
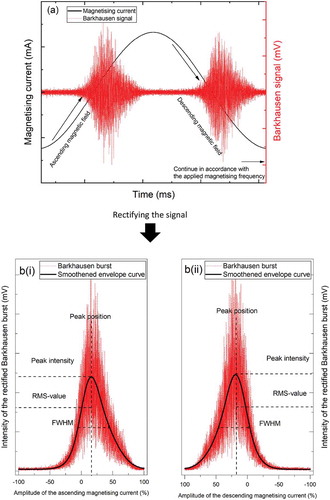
Figure 2. Data from process monitoring of induction-hardened case depth of the cam lobes by means of MBN method
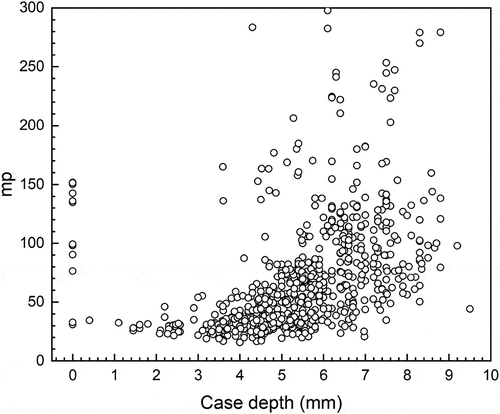
Table 1. Chemical composition of the DIN 17,212 grade CF53N carbon steel
Figure 3. A schematic diagram shows the Barkhausen measurements (a) in the production line and (b) in the laboratory
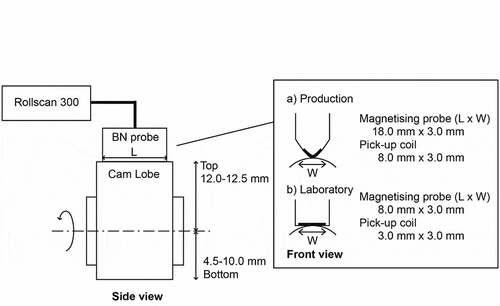
Figure 4. Cross-sectional metallography of a qualified induction-hardened component: (a) an optical micrograph; (b) the uppermost martensite layer (0.0 – ~1.0 mm deep); (c) the mixed martensite and bainite in the upper alternating layer (~2.0 mm deep); (d) the mixed bainite, pearlite, martensite and ferrite in the low alternating layer (~4.0 mm deep); (e) the pearlite-ferrite inner core (~5.0 mm deep)

Figure 5. The smoothened envelopes of the rectified Barkhausen bursts obtained during (a) ascending magnetic field excitation and (b) descending magnetic field excitation of the CF53N steel in different heat-treated forms, i.e. pure martensite (Sample A), the bainite-pearlite-martensite-ferrite mixture (Sample B) and the pearlite-ferrite mixture (Sample C)
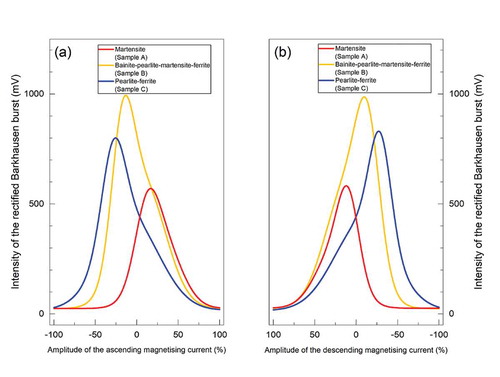
Figure 6. Pulse height distribution of the MBN signals from pure martensite (Sample A), the mixed bainite-pearlite-martensite-ferrite (Sample B) and the mixed pearlite-ferrite (Sample C)
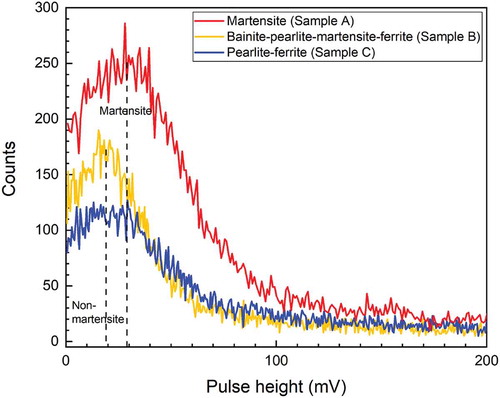
Figure 7. Amplitude spectra from the MBN measurements from pure martensite (Sample A), the mixed bainite-pearlite-martensite-ferrite (Sample B) and the mixed pearlite-ferrite (Sample C)
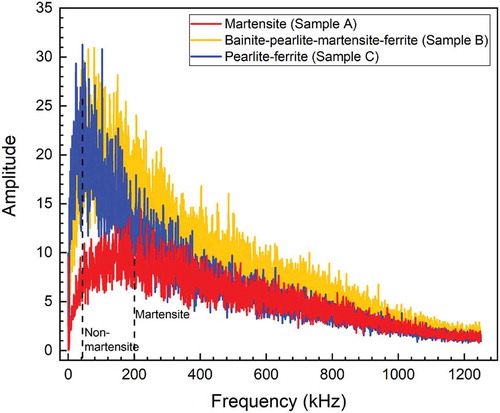
Table 2. Classification of different surface conditions after the induction hardening process
Table 3. Parametric features of the Barkhausen measurements from different induction-hardened surface conditions
Figure 8. Plots of case depth vs. (a) mp and (b) the peak position of the Barkhausen burst stratify on microstructural classifications
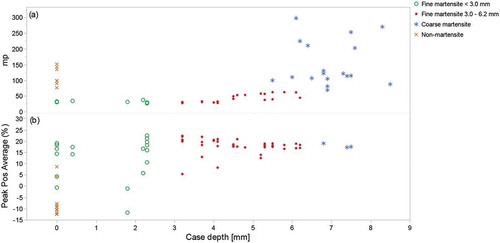
Table 4. Decision criteria (DC) based on the Barkhausen burst characteristics for induction-hardened surface classification
Figure 9. Data splitted into two random sub-sets, i.e. training (75 %) and validation (25 %), for statistical modelling
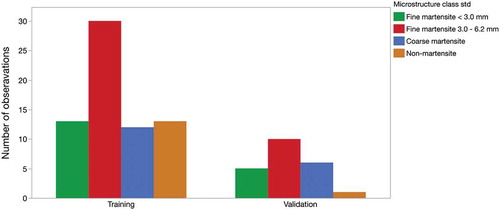
Figure 10. Results of the regression decision tree analysis using the partition platform in JMP Pro®. (a) A statistical overview after 7 splits; (b) summary of the decision tree model; (c) statistical figures of each branch in the decision tree model; (d) a predictive plot of the induction-hardened case depth based on the decision tree model
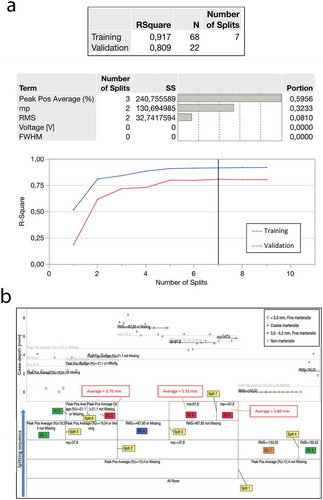