Figures & data
Table 1. Representative radioisotopes for PET imaging and their production methods.
Figure 1. (a) Preparation of PET nanoprobes targeted at αvβ3 integrin. (b) Noninvasive PET/CT images of angiogenesis induced by hindlimb ischemia in amurine model for nontargeted dendritic nanoprobes (shown bottom center). (c) Noninvasive PET/CT images of angiogenesis induced by hindlimb ischemia in a murine model for αvβ3-targeted dendritic nanoprobes, which showed higher uptake in ischemic hindlimb (left side of image) as compared with control hindlimb (right side of image) (adapted from Almutairi et al., Citation2009).
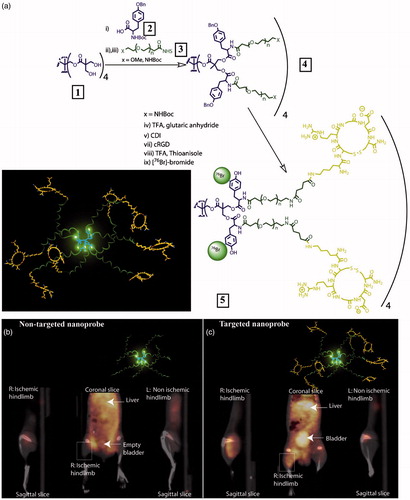
Figure 2. (a) Schematic structure of 64Cu-DPCZ which is constituted by four components, PAMAM G0 as a scaffold, Cy5.5 as an optical reporter, 64Cu-DOTA as a PET reporter and Affibody as a tumor-targeting molecule; (b) In vivo NIRF imaging of SKOV3 tumor-bearing mice at 0.1, 1, 2, 4, 8 and 20 h after tail vein injection of 64Cu-DPCZ; (c) Decay-corrected coronal micro-PET images of mice bearing SKOV3 tumor at 1, 2, 4 and 20 h after tail vein injection of 64Cu-DPCZ. Arrows indicate the location of the tumors (n = 3) (adapted from Wang et al., Citation2014).
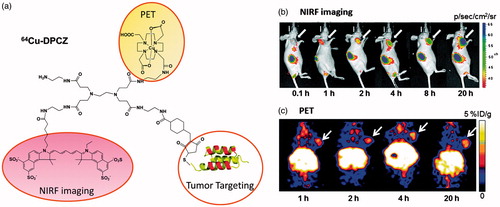
Figure 3. Nanoporphyrin-mediated MRI and PET imaging in animal models. (a) In vitro MRI signal of Gd-NPs in the absence and in the presence of SDS obtained by T1-weighted MRI on a Bruker Biospec 7 T MRI scanner using a FLASH sequence. (b) Representative coronal and axial MR images of transgenic mice with mammary cancer (FVB/n Tg(MMTV-PyVmT) using a FLASH sequence preinjection and after injection of 0.15 ml Gd-NPs (Gd dose: 0.015 mmolkg−1). The white arrow points to the tumor site. (c) PET image of nude mice bearing SKOV3 ovarian cancer xenografts at 4-, 8-, 16-, 24- and 48-h post-injection of 64Cu-labeled NPs (150–200 μl, 64Cu dose: 0.6–0.8 mCi). The white arrow points to the tumor site. (d) 3 D coronal MR images of nude mice bearing A549 lung cancer xenografts using a FLASH sequence at 4- or 24-h post-injection with 0.15 ml of 64Cu and Gd dual-labeled NPs (150–200 ml, 64Cu dose: 0.6–0.8 mCi, Gd dose: 0.015 mmol kg−1). The white arrow points to the tumor site. (e) PET-MR images of tumor slices of nude mice bearing A549 lung cancer xenograft at 4- or 24-h post-injection of dual-labeled NPs. White arrow points to the necrotic area in the center of the tumor (adapted from Li et al., Citation2014).
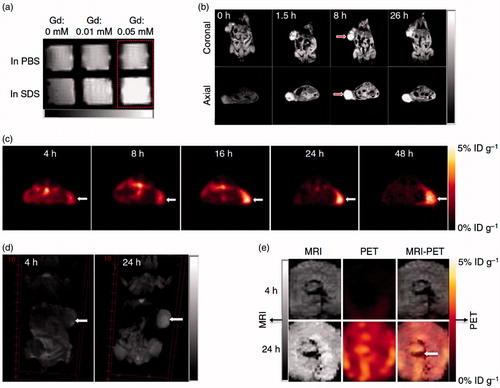
Figure 4. Schematic representation of a new approach for pretargeted PET imaging that leverages the utilities of supramolecular nanoparticles (SNPs) and bioorthogonal chemistry: (a) Supramolecular synthetic strategy is employed for preparing the tumor-targeting agent (TCO⊂SNPs); (b) after intravenous injection, the tumor EPR effect drives preferential accumulation of TCO⊂SNPs in tumor; (c) after TCO⊂SNPs have accumulated in tumor, TCO⊂SNPs disassemble to release a TCO-grafted molecular building block, TCO/CD-PEI; (d) a radiolabeled reporter (64Cu-Tz) is then injected for bioorthogonal reaction with tumor-retained TCO/CD-PEI; (e) the unreacted 64Cu-Tz was cleared quickly from the body; (f) the resulting dihydropyrazine (DHP) conjugation adduct (64Cu-DHP/CD-PEI) confines radioactivity in tumor, resulting in high-contrast tumor PET imaging. (g) Chemical structures of the bioorthogonal reactions between TCO/CD-PEI and 64Cu-Tz (adapted from Hou et al., Citation2016).
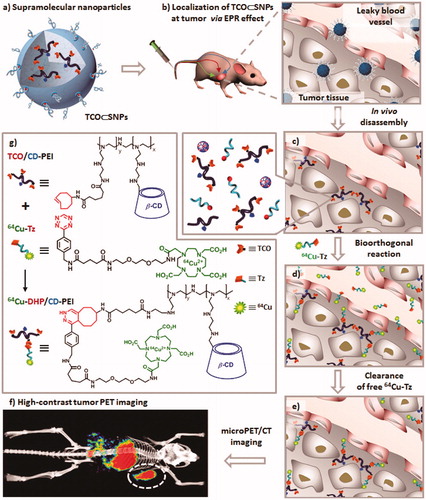
Figure 5. Generation and structure of N-glycan (bis-Neua(2-6)Galglycan (a), bis-Neua(2-6)Gal-asialo glycan (b) and bis-Neua(2-3)Galglycan (c)) clusters labeled with 68Ga-DOTA (adapted from van Ghobril et al., Citation2012).
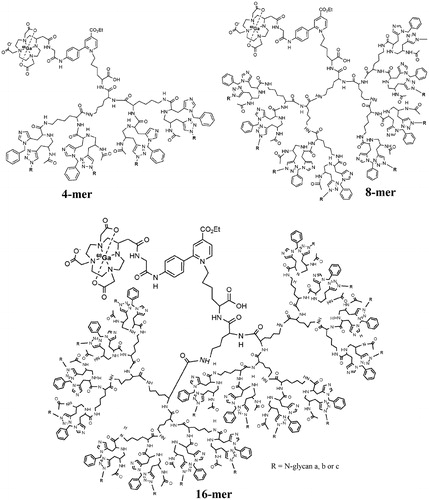