Figures & data
Figure 1. Expression of surface markers on mural cells. (A) Expression of PDGFRβ, NG2, and α-SMA in pericytes (PCs), smooth muscle cells (SMCs), and endothelial cells (ECs). (B) Co-localization of PDGFRβ, NG2, or α-SMA with CD31 in tumor tissues derived from LS174T tumor grafts. The nuclei were visualized by DAPI staining. Original magnification 200×.
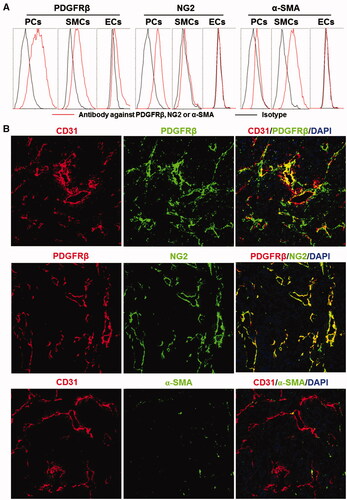
Figure 2. Preparation and characterization of the ZPDGFRβ affibody. (A) SDS-PAGE and size-exclusion chromatography of the purified ZPDGFRβ affibody in the presence or absence of 2-ME. (B) Binding of the ZPDGFRβ affibody to pericytes (PCs), smooth muscle cells (SMCs), and endothelial cells (ECs) as analyzed by flow cytometry. (C) Inhibition of pericyte binding of the ZPDGFRβ affibody by the PDGFRβ-specific antibody (left) and the PDGFRβ-binding of the ZPDGFRβ affibody analyzed using the OpenSPR system (right). (D) Cytotoxicity of the ZPDGFRβ affibody in pericytes (PCs), smooth muscle cells (SMCs), and endothelial cells (ECs).
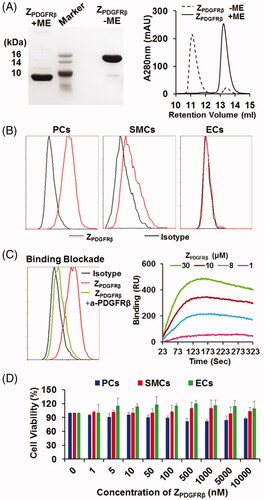
Figure 3. Tumor-homing characteristics of the ZPDGFRβ affibody. (A) Tumor uptake of the ZPDGFRβ affibody. CF750-labeled ZPDGFRβ affibody was intravenously injected into mice (n = 3) bearing subcutaneous LS174T tumor grafts (arrow indicated), followed by scanning with SPECTRAL Lago and Lago X Imaging Systems at different times (0–4 h) post-injection. (B) Bio-distribution of the ZPDGFRβ affibody in mice bearing LS174T tumor grafts. Mice (n = 3) injected with CF750-labeled ZPDGFRβ affibody were sacrificed at 4 h post-injection for optical imaging of organs/tissues. (C) Co-localization of the ZPDGFRβ affibody with CD31, PDGFRβ, or NG2 in tumor tissues. FAM-labeled ZPDGFRβ affibody was intravenously injected into mice bearing LS174T tumor grafts. Tumor grafts were removed and sectioned under freezing conditions, followed by staining with antibody against CD31, PDGFRβ, or NG2. The nuclei were visualized by DAPI staining. Original magnification 200×.
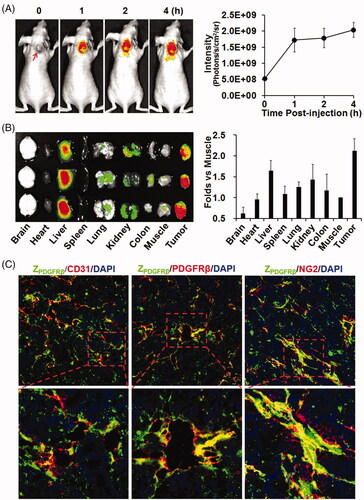
Figure 4. ZIR700-mediated PDT kills PDGFRβ-expressing cells in vitro. (A) Flow cytometry analysis of PDGFRβ expression (left) and ZIR700 binding (right) to pericytes (PCs) and LS174T tumor cells. (B) Cytotoxicity of ZIR700 in PDGFRβ-expressing pericytes under different conditions. Cells were pre-incubated with ZIR700 for 1 h followed by washing with PBS and illumination with (ZIR700+Light) or without (ZIR700-Light) laser. The live/dead cells were visualized by SYTO9/PI staining. Original magnification 200×. (C) Production of ROS in pericytes treated with ZIR700-mediated PDT. Cells were incubated with DCFH or SOSG during ZIR700-mediated PDT and were observed under a fluorescence microscope (left) or measured with a fluorescence microplate reader (right). Original magnification 200×.
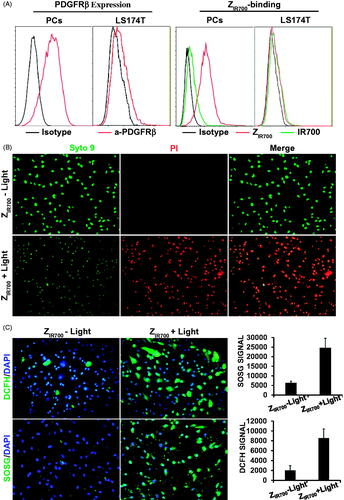
Figure 5. ZIR700-mediated PDT suppresses LS174T tumor growth in mice. (A) Permeability of tumor blood vessels visualized by the leakage of dextran. After intravenous injection of ZIR700 followed by illumination (ZIR700 + Light) or not (ZIR700−Light), mice bearing LS174T tumor grafts were intravenously injected with FITC-dextran at different times (0–8 h) post-illumination. Approximately 20 min later, the tumor grafts were removed and sectioned under freezing conditions, followed by visualization of the blood vessels with antibody against CD31. DAPI was used to visualize the nuclei. Original magnification 100×. (B) Expression of HIF1α in tumor tissues at different times (1–8 h) post-illumination. Original magnification 200×. (C) H&E staining of tumor tissues derived from tumor grafts at different times (0–72 h) post-illumination. (D) Tumor growth suppression by ZIR700-mediated PDT. Mice (n = 3) bearing LS174T tumor grafts were intravenously injected with ZIR700, followed by illumination (ZIR700+Light) or not (ZIR700-Light) at day 0 and day 3, respectively. Mice in the control group were treated with PBS. The tumor volumes were measured every day, and the (1–3) growth curves of mice in each group are shown. At the end of this experiment, all tumor grafts were removed and weighed.
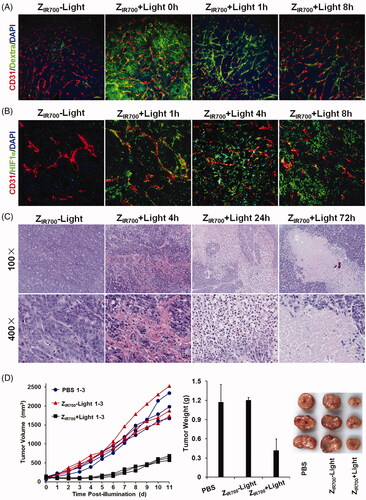
Figure 6. ZIR700-mediated PDT and TRAIL combination therapy leads to a better antitumor effect. (A) ZIR700-mediated PDT increases the tumor uptake of TRAIL. Mice bearing LS174T tumor grafts were treated with FAM-labeled TRAIL (TRAIL) or ZIR700 combined with TRAIL followed (ZIR700/TRAIL + Light) or not followed by (ZIR700/TRAIL-Light) illumination. The tumor grafts were removed and sectioned under freezing conditions 1 h post-injection of TRAIL. The blood vessels were visualized with antibody against CD31. The nuclei were visualized by DAPI staining. Original magnification 200×. (B) ZIR700-mediated PDT and TRAIL combination therapy induced stronger apoptosis. Mice bearing LS174T tumor grafts were treated with ZIR700-mediated PDT alone (ZIR700+Light) or ZIR700 combined with TRAIL followed (ZIR700/TRAIL + Light) or not followed (ZIR700/TRAIL-Light) by illumination. Tumor grafts were removed 16 h post-injection of TRAIL and sectioned under freezing conditions. TUNEL was used to visualize apoptotic cells in tumor tissues. Original magnification 200×. (C) ZIR700-mediated PDT and TRAIL combination therapy shows greater tumor growth suppression. Mice (n = 6–7) were treated with TRAIL or ZIR700-mediated PDT (ZIR700+Light) or TRAIL and ZIR700-mediated PDT combination therapy (ZIR700/TRAIL + Light). PBS was used as a control.
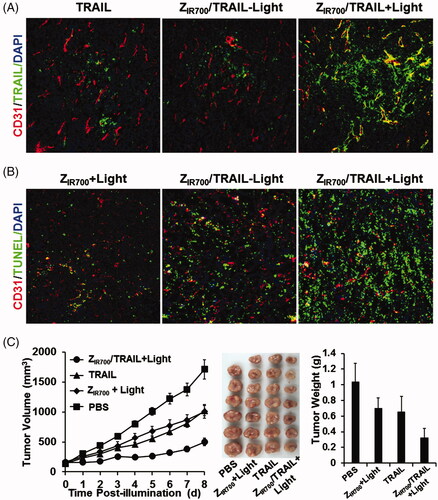