Figures & data
Figure 1. (A, B) Pneumatic pulsed pressure bioreactor composed of a (1) 13 N·m closed-loop bipolar stepper motor with a (2) 40 mm radius crankshaft mechanism driving a (3) 50 mm bore 157 ml pneumatic actuator piston with an 80 mm stroke. (C) The linear piston displacement was calculated using (t)≈r(1 − cos(θ(t))) with a resolution of 0.36°/step at the motor. (D) Three components were designed and machined in stainless steel to enable a robust mechanical connection between the stepper motor and the pneumatic piston, comprising a (2a) crankshaft, (2 b) a linkage rod, and (2c) a screw attachment which directly affixed to the piston actuator rod. (E) The bioreactor was driven by a closed-loop stepper driver and controlled by an Arduino UNO microcontroller, whilst a real-time user interface was created in C# for Windows for control over experimental variables (pressure frequency and maximum load, duration/number of cycles) via USB to the Arduino. A 400 kPa analogue sensor was used to provide real-time feedback of the pressure created in the chamber, and a Hall effect sensor used to control positional accuracy of the shaft. (F) When tested, the bioreactor was capable of outputting pulses of air from the cylinder at frequencies up to 3.5 Hz and pressures of up to 400 kPa. Scale bar is 10 cm.
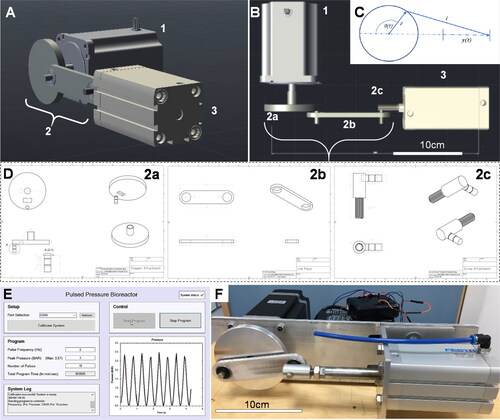
Table 1. Overall design parameters for the bioreactor.
Figure 2. Parameterization of the bioreactor. Determining the operational parameters involved establishing the relationship between the compressible air volume in the system, which was a variable based on the liquid/cell volume occupying the cell culture chamber, and the mechanical moment applied by the motor-piston assembly. (A) The relationship between maximum chamber pressure and spare volume in the system was calculated and plotted. (B) The maximum achievable chamber pressure and resulting torque/moment acting on the motor shaft through a full rotation was calculated based on attachment of the apparatus to an empty 57.3 ml bioreactor chamber.
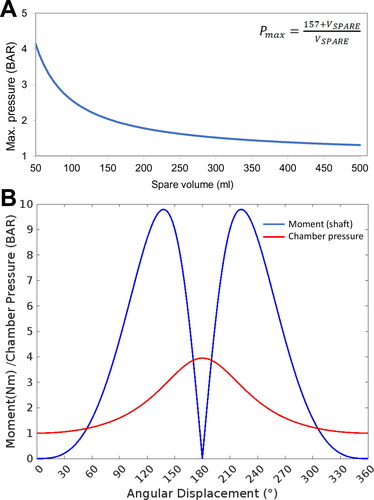
Figure 3. The 3 D printed cell culture bioreactor pressure chamber. (A) A 3 D printed bioreactor culture chamber was designed with a removable sliding tray to hold (B) a standard transwell insert containing (C) microspheres of tissue engineered cartilage which could be assembled in a sterile flow hood and made gas-tight using four simple bolts and a 4 mm neoprene O-ring as a gasket. The chamber was (D) connected to the main bioreactor pressure outlet via a pressure hose with an inline sterile 25 mm (0.4 μm) syringe filter to sterilize the input air which fitted into a recess on the inside of the chamber front. The scale bars in Figure 3A, B, and D are 1 cm and 3 mm in Figure 3C.
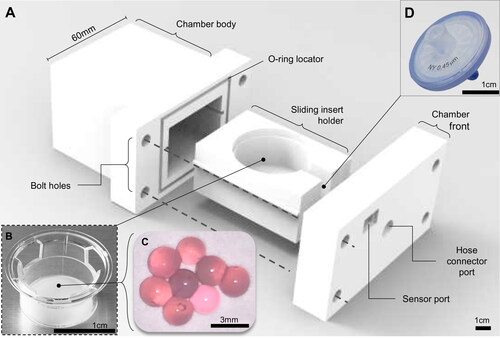
Figure 4. Biological effects of dynamic pressure on chondrocytes in alginate-based hydrogels. (A) Chondrocyte proliferation/metabolic activity (measured by MTT assay) was increased by 21% after 5 days of loading in the bioreactor. (B) Extracellular matrix (ECM) composition analysis. Both total protein and glycosaminoglycan production by chondrocytes were quantified as biomarkers of cartilage growth. The glycosaminoglycan content in the engineered tissues was significantly increased in response to pulsed pressure. Statistical analysis was performed using Student’s t-test (n = 14-18).
** indicates p < 0.01.
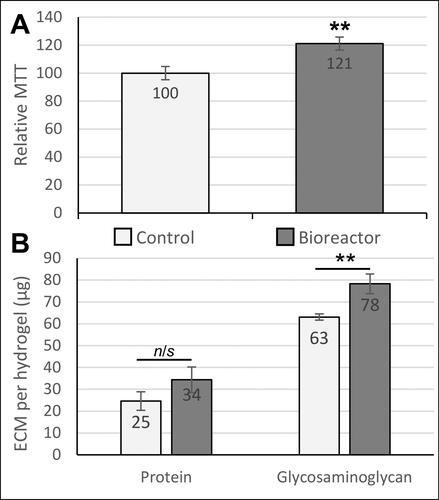