Figures & data
Figure 1. The hallmarks of aging. These key factors represent a framework proposed to comprehensively describe the complex processes that contribute to aging at the cellular and molecular levels. The hallmarks include: Loss of proteostasis (decline in the cellular machinery responsible for maintaining protein homeostasis); Mitochondrial dysfunction (deterioration in mitochondrial function, including reduced energy production and increased generation of reactive oxygen species); Stem cell exhaustion (reduced regenerative capacity due to the depletion or dysfunction of stem cells); Cellular senescence (accumulation of cells that have ceased dividing and often secreting pro-inflammatory molecules); Altered intercellular communication (changes in the communication between cells, leading to the chronic low-grade inflammation known as inflammaging); Genomic instability (accumulation of DNA damage over time, leading to mutations); Telomere attrition (shortening of telomeres with each cell division); Deregulated nutrient sensing (impaired metabolic function as a result of dysregulation of nutrient-sensing signaling pathways). According to the prevailing opinion, one of the identified hallmarks of aging - Еpigenetic changes (alterations in epigenetic marks, such as DNA methylation and histone modifications) most likely has a leading role and controls the aging processes. Modified from López-Otín et al. [Citation2].
![Figure 1. The hallmarks of aging. These key factors represent a framework proposed to comprehensively describe the complex processes that contribute to aging at the cellular and molecular levels. The hallmarks include: Loss of proteostasis (decline in the cellular machinery responsible for maintaining protein homeostasis); Mitochondrial dysfunction (deterioration in mitochondrial function, including reduced energy production and increased generation of reactive oxygen species); Stem cell exhaustion (reduced regenerative capacity due to the depletion or dysfunction of stem cells); Cellular senescence (accumulation of cells that have ceased dividing and often secreting pro-inflammatory molecules); Altered intercellular communication (changes in the communication between cells, leading to the chronic low-grade inflammation known as inflammaging); Genomic instability (accumulation of DNA damage over time, leading to mutations); Telomere attrition (shortening of telomeres with each cell division); Deregulated nutrient sensing (impaired metabolic function as a result of dysregulation of nutrient-sensing signaling pathways). According to the prevailing opinion, one of the identified hallmarks of aging - Еpigenetic changes (alterations in epigenetic marks, such as DNA methylation and histone modifications) most likely has a leading role and controls the aging processes. Modified from López-Otín et al. [Citation2].](/cms/asset/2e3fef19-ed1e-4b9c-a137-fabbc2c1ce9a/tbeq_a_2358999_f0001_c.jpg)
Figure 2. Cell reprogramming stages. Reprogramming of differentiated cells (blue cell) with a specific combination of transcription factors: Oct4, Sox2, Klf4 and c-Myc (OSKM) leads to the induction of pluripotency and acquiring the characteristics of pluripotent stem cell (red cell). During the reprogramming process, the cell passes through several stages including changes in DNA methylation patterns and histone modifications. This reshapes the cell’s epigenetic landscape but still leaves specific somatic genes expressed (round green cell). If the reprogramming continues, the resulting changes would lead to the activation of the pluripotent genes and, after their stabilization, the cells will become induced pluripotent cells (red cell). If the effects of Yamanaka factors are terminated before the activation of pluripotent genes, the cell can stabilize in a rejuvenated state (green cell) without losing its somatic identity. Cells in the blue box represent intermediate stages during reprogramming.
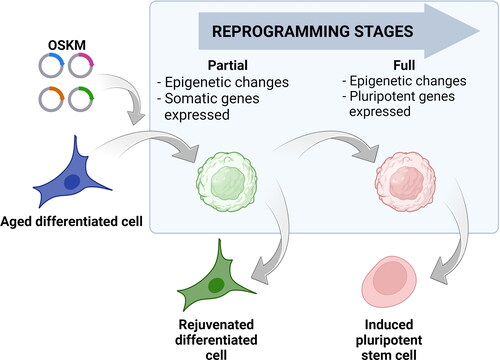
Figure 3. Potential applications of membrane potential change in cell reprogramming. Yellow box: Since hyperpolarization is associated with the differentiated cell state, there is a possibility that the experimental increase in membrane potential can be used to slow down and even stop the process of cellular reprogramming carried out with Yamanaka factors. Such ‘stretching’ of the process creates an opportunity to stabilize cells before the activation of pluripotency genes, but after the already occurring epigenetic changes ensuring cellular rejuvenation. Green box: Conversely, forced membrane depolarization and following repolarization of aged differentiated cells may be an alternative to the transfection-induced approach for partial reprogramming. Because lowering the membrane potential by manipulating ion concentration or activity of ion channels are able to delay or block or potentially revert differentiation, there is a possibility that this approach could prove to be an effective way of cell rejuvenation.
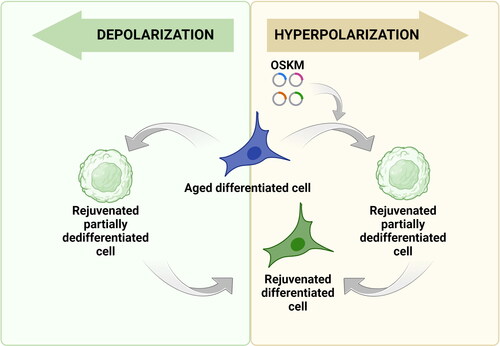
Data availability
Data sharing is not applicable to this article as no new data were created or analyzed in this study.