Figures & data
Figure 1. Ropes in an elevator shaft. The compensation rope connects the car and the counterweight on the lower side via the compensating sheave for balancing the mass.
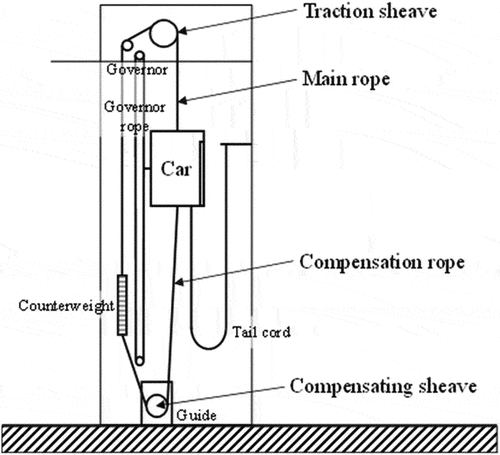
Figure 3. Sinusoidal curve y = a sin x () and its length l. When a straight line of length l bends in the shape of a sin x, the right edge moves to the left by l-π.
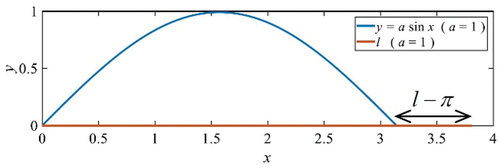
Figure 4. Applicable range of approximate curve. The relation between a and l-π differs depending on whether a is small or large. (a) (0, 0.1]; (b) (0, 10.0].
![Figure 4. Applicable range of approximate curve. The relation between a and l-π differs depending on whether a is small or large. (a) (0, 0.1]; (b) (0, 10.0].](/cms/asset/9c0cc235-dd18-4e6c-b96f-29689213d76c/tabe_a_2086876_f0004_oc.jpg)
Table 1. Approximate curves.
Figure 5. Relation between vertical vibration of the compensating sheave z and horizontal vibration of the compensation rope a.
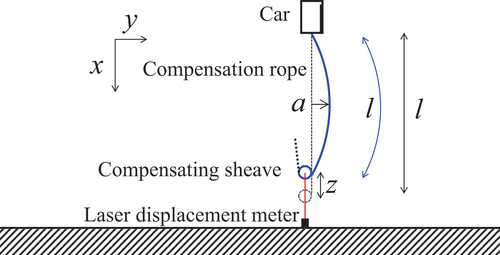
Figure 6. Rope length l when the vertical displacement of the compensating sheave is z and the horizontal amplitude of the compensation rope is a. (b) is the view of (a) from the upper side of the l-axis.
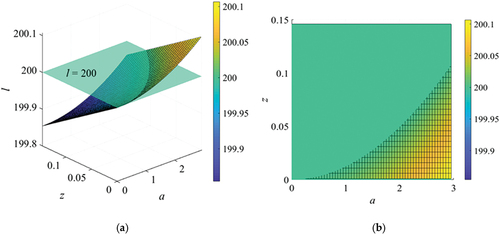
Table 2. Approximate formulae of vertical displacement z. a varies from 0.005 to 2.495 in increments of 0.01. The coefficient of the first-order term increases when the rope length l becomes small.
Figure 7. Vertical vibration of the compensating sheave zN and horizontal vibration of the compensation rope aN for each mode. (a) 1st; (b) 2nd; (c) 3rd; (d) Combination of 1st to 3rd modes (a1: a2: a3 = 5: 3: 2).
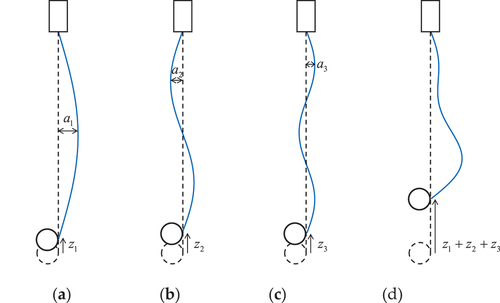
Figure 9. Rope tension distribution and mode shape distortion. (a) Rope tension distribution; (b) mode shape.
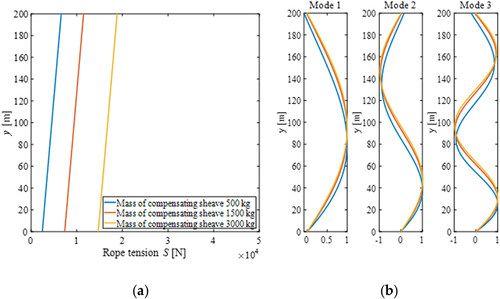
Table 3. Natural frequencies of the building (1st to 5th order).
Table 4. Parameters of the analysis model.
Figure 10. Acceleration records resized according to the peak ground velocity of 0.25 m/s, their Fourier amplitude spectra, and displacement disturbance input to the rope. (a) Heisei 16 Niigata Prefecture Chuetsu Earthquake observed in Shinjuku (EW(East-West) component); (b) 2011 Tohoku Earthquake off the Pacific coast observed in Konohana (EW component).
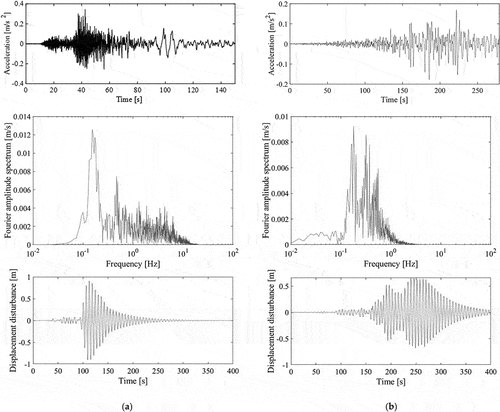
Figure 12. Vertical displacement of the compensating sheave. (a) Heisei 16 Niigata Prefecture Chuetsu Earthquake; (b) 2011 Tohoku Earthquake off the Pacific coast.

Figure 13. Modal displacements. Five modes are used for numerical analysis, but the responses after the third order are very small. (a) Heisei 16 Niigata Prefecture Chuetsu Earthquake; (b) 2011 Tohoku Earthquake off the Pacific coast.

Figure 14. Coefficient of EquationEquation (17)(17)
(17) (N0 = 3). The sum of the three values is 1. (a) Heisei 16 Niigata Prefecture Chuetsu Earthquake; (b) 2011 Tohoku Earthquake off the Pacific coast.
