Figures & data
Figure 1. The considered initial configuration ℐ of the three substances in the microscopic pore-space μ, where . The grain μ is located in the centre of Y and the pore-space μ is occupied by the two immiscible and incompressible fluids P1 (water) and P2 (oil) as well as the precipitate P2. The spherical perforated precipitate P3 is attached to the boundary Гμ of the grain μ, the spherical fluid P2 domain is located in the corners of the microscopic domain Y and the fluid P1 occupies the remainder.
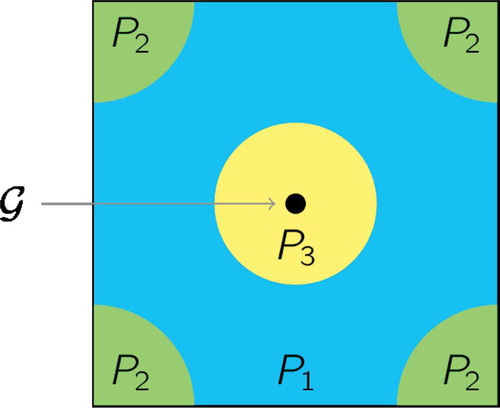
Table 1. The varying parameters of the three settings S1, S2 and S3, which are explained in Section 4.1 and . The function u_(t) = a:b increases linearly from a to b with the time-steps in the course of a simulation. For i = 1,2 an initial precipitate’s radius results in a precipitate’s initial portion of
on the overall pore-space’s volume, analogously the water’s initial portion is
, and the oil’s initial portion is
due to a radius
.
Figure 2. The initial phase configurations in the microscopic pore-space in the two subdomains
and
of the macroscopic domain
. The black points in the very centre of
in (b) and (c) represent the small spherical grains
. Each grain is surrounded by a precipitate
(yellow). For
,
defines the initial radius of the precipitates in the subdomain
. Each precipitate is surrounded by water (blue) and a spherical oil phase
(green) is located in the corners of
. The coloured subdomains Pi are characterized by
for
. Full colour available online.
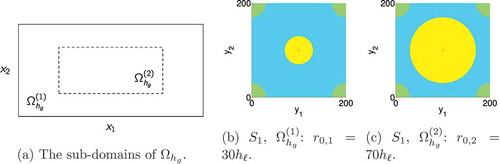
Figure 3. The nodes in the subset of the macroscopic grid
: nodes of
are indicated in red, and in blue those of
. Full colour available online.
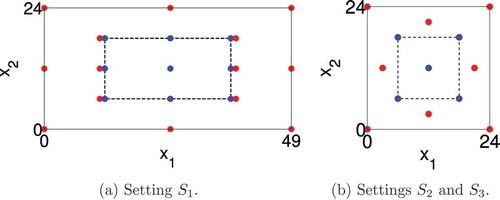
Figure 5. High-dimensional solution of the setting : the evolution of the precipitates’ volume portion on the pore-space in the macroscopic nodes
(red dashed),
(blue dotted),
(black) and
(cyan). In
the node
(and respectively,
) is host of the precipitate with the minimum (maximum) volume portion throughout the time-interval. The same relation holds in
for
(minimum) and
(maximum). Full colour available online.
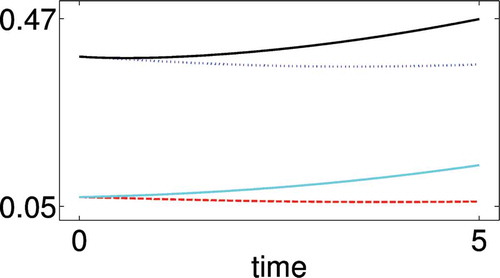
Table 2. Results for setting : the column entitled by ‘speed-up’ presents the rough speed-up of the approximations’ computation time in comparison to the high-dimensional solution of
in 57 h (as for the high-dimensional solution
processors of type Intel(R) Xeon(R) CPU [email protected] GHz were used), and the columns entitled by
,
,
and
present the approximations’ accuracy. The line entitled by
presents the results of a high-dimensional approximation with prescribed
resulting in
and
.
Table 3. Results for setting : the columns entitled by
,
and
present the average sizes of the
basis sets of a reduced model, and the column entitled by ‘reduction time’ presents the time that one processor of type Intel(R) Xeon(R) CPU [email protected] GHz needs to construct the reduced models from the pre-computed snapshots of the high-dimensional solution of
. In particular, this time is not the full offline time, as it does not include the 57-h for the snapshots computation.
Figure 6. Results for the setting and the low-dimensional solution
with
: (a) presents the evolution of the precipitates’ volumes
and (b) the utilized basis sets in the course of the simulation in the macroscopic nodes
(red dashed),
(blue dotted),
(black) and
(cyan). Full colour available online.
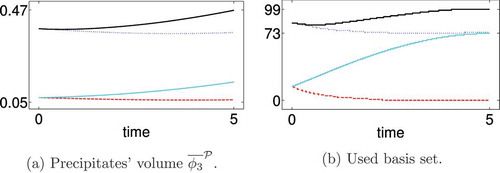
Figure 7. Results for the setting and the low-dimensional solution
: (a) depicts the minimum (black) and maximum (cyan) of the volume interval for the precipitates for the
basis sets that are used in the online phase to determine the proper basis set in order to approximate the reduced solution of
, where the minimum is
for basis set
and the maximum is
for basis set
; (b) shows the number of POD modes (cyan) and the number of magic points (black) of the basis sets and (c) depicts exemplary for the basis set
for the increasing number of modes the decay of
of the eigenvalues of the correlation operator in the construction of the POD basis (cyan) and for the increasing number of magic points the error decay of the EI (black). Full colour available online.
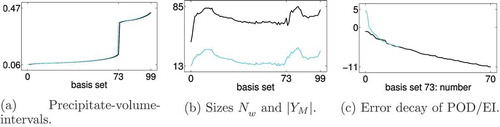
Figure 8. Results for setting and the low-dimensional solution
, which consists of
basis sets: (a), (b) and (c) illustrates the distribution of the magic points in the basis sets
,
and
, where the inner circle marks the smallest and the outer one the largest subdomain supporting the precipitate in the snapshots, and the four quarters of a circle indicate the almost unchanged oil phase; (d), (e) and (f) depict the dominant POD mode for
for the corresponding basis sets.
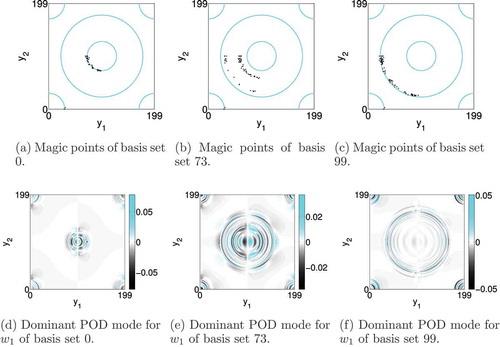
Table 4. Setting S2: the approximations’ accuracy.
Table 5. Setting S3: the approximations’ accuracy.