Figures & data
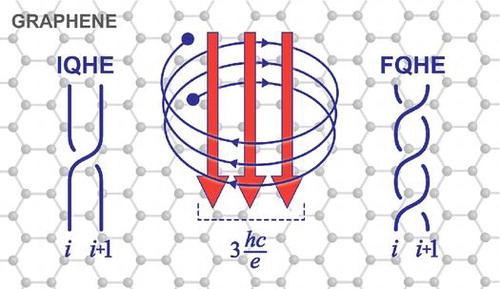
Figure 1. Schematic demonstration that commensurability (left) of cyclotron orbit with interparticle separation satisfies topology requirements for braid interchanges in uniformly and equidistantly (due to interaction) distributed 2D particles; for smaller cyclotron radii particles cannot be matched (center), for larger ones the interparticle distance cannot be conserved (right).
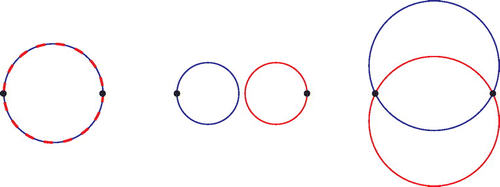
Figure 2. Schematic illustration of cyclotron orbit enhancement in 2D due to multi-loop trajectory structure (third dimension added for visual clarity).
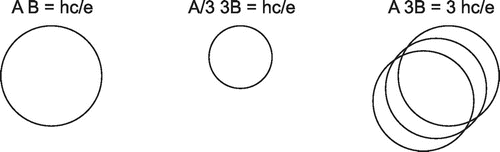
Figure 3. The braid generator corresponds to the single exchange of particles (left), the cyclotron orbit (relative) corresponds to the double exchange (right), for (a)
when single-looped cyclotron trajectory reaches neighboring particles,
; and (b) braid generator
for
with additional loop needed for
(
is cyclotron radius,
is particle separation, i.e.
,
).
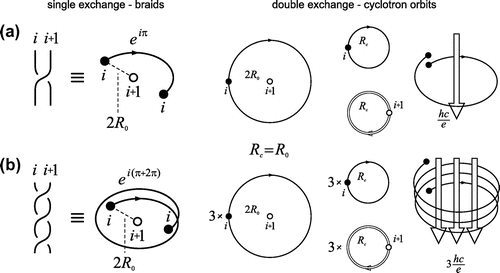
Figure 4. Evolution of fractional filling hierarchy in three first LLs of the monolayer graphene; for each LL the first particle subband is illustrated, the next subbands in each LL repeat the hierarchy from the first one. Different types of ordering are indicated with spikes of various height. Series for ordinary FQHE(multiloop), FQHE(single-loop), Hall metal and paired state are displayed according to the hierarchy described in Table with ,
; only a few selected ratios from these series are explicitly written out.
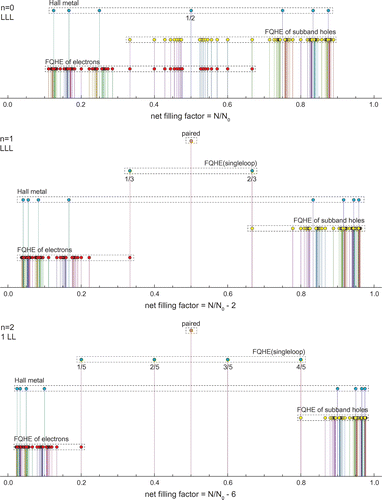
Table 1. LL filling factors for FQHE determined by commensurability condition (paired indicates condensate of electron pairs), for the first particle subband in each of the three first LLs () for the monolayer graphene.; subb. stands for subband.
Table 2. LL filling factors for FQHE determined by commensurability condition (paired indicates condensate of electron pairs), for the first particle subband in each of the two first LLs ( for the extra degenerated LLL and
for the first LL beyond the LLL) for the bilayer graphene.
Table 3. Comparison of filling hierarchy in the LLL level in the bilayer graphene for two mutually inverted successions of two lowest subbands: ,
(upper) and
,
(lower).
Figure 5. Evolution of fractional filling hierarchy in the two first LLs of the bilayer graphene; for the LLL two subbands with and
are illustrated. Different types of ordering are indicated with spikes of various heights. Series for ordinary FQHE(multiloop), FQHE(single-loop), Hall metal and paired state are displayed according to the hierarchy described in Table with
,
; only a few selected ratios from these series are explicitly written out.
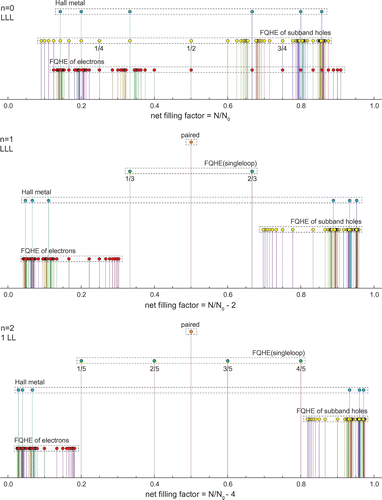
Figure 6. (a) FQHE observation in suspended graphene for the filling 0.3 (1 / 3) in a field of 12–14 T with the concentration of cm
and the mobility of 250,000 cm
V
s
; (b) FQHE singularities in suspended graphene for the filling
in a field of 2–12 T with the concentration of
cm
and the mobility of 200,000 cm
V
s
(after [Citation28,Citation29]).
![Figure 6. (a) FQHE observation in suspended graphene for the filling 0.3 (1 / 3) in a field of 12–14 T with the concentration of 1011 cm-2 and the mobility of 250,000 cm2V-1s-1; (b) FQHE singularities in suspended graphene for the filling 13 in a field of 2–12 T with the concentration of 1010 cm-2 and the mobility of 200,000 cm2V-1s-1 (after [Citation28,Citation29]).](/cms/asset/f07291a4-3294-4e35-8bce-be4fc4a80776/tsta_a_1145531_f0006_oc.gif)
Figure 7. The emergence of an insulator state accompanying the increase in the strength of a magnetic field around the Dirac point; (b) competition between FQHE and the insulator state for the filling : annealing removes pollution – enhances mobility and provides conditions for the emergence of plateau for FQHE (after [Citation29]).
![Figure 7. The emergence of an insulator state accompanying the increase in the strength of a magnetic field around the Dirac point; (b) competition between FQHE and the insulator state for the filling -1/3: annealing removes pollution – enhances mobility and provides conditions for the emergence of plateau for FQHE (after [Citation29]).](/cms/asset/40ae082a-386b-43a6-b4b3-7f6c95e756cf/tsta_a_1145531_f0007_oc.gif)
Figure 8. Fractional quantum Hall effect for graphene on BN. Magnetoresistance (left axis) and Hall conductivity (right axis) in the and
Landau levels at
T and temperature
K (after [Citation4]). All filling ratios indicated in blue agree with the hierarchy given in Table .
![Figure 8. Fractional quantum Hall effect for graphene on BN. Magnetoresistance (left axis) and Hall conductivity (right axis) in the n=0 and n=1 Landau levels at B=35 T and temperature ∼0.3 K (after [Citation4]). All filling ratios indicated in blue agree with the hierarchy given in Table 1.](/cms/asset/db3002a9-f1ba-4bb1-94c7-63f7ae5a8c02/tsta_a_1145531_f0008_oc.gif)
Figure 9. Magnetoresistance (left axis) and Hall resistance for graphene on BN (right axis) versus gate voltage acquired at B = 35 T. Inset shows Shubnikov-de Haas oscillations at Vg = –18.5 V (after [Citation4]). All filling ratios indicated in the figure (in blue) agree with the hierarchy given in Table .
![Figure 9. Magnetoresistance (left axis) and Hall resistance for graphene on BN (right axis) versus gate voltage acquired at B = 35 T. Inset shows Shubnikov-de Haas oscillations at Vg = –18.5 V (after [Citation4]). All filling ratios indicated in the figure (in blue) agree with the hierarchy given in Table 1.](/cms/asset/0cfb8e92-2fbb-46e3-8693-c373af2b9c3b/tsta_a_1145531_f0009_oc.gif)
Figure 10. Fan diagram for for graphene up to 11 T (after [Citation5]).
![Figure 10. Fan diagram for ρxx(ν,B) for graphene up to 11 T (after [Citation5]).](/cms/asset/2efa3bb8-8720-476a-8d80-23c4e0e633f3/tsta_a_1145531_f0010_oc.gif)
Figure 11. Not fully developed FQHE states with residual longitudinal resistance, corresponding to correlation of every second or every third particles at fractional rates reproduced by the commensurability series with
(upper panels visualize longitudinal resistivity measurements after [Citation5]).
![Figure 11. Not fully developed FQHE states with residual longitudinal resistance, corresponding to correlation of every second or every third particles at fractional rates reproduced by the commensurability series ν=2(3,4)+xll3(q-1)±1 with q=3,x=2,3,l=i3,i=1,2,3 (upper panels visualize longitudinal resistivity measurements after [Citation5]).](/cms/asset/cb39a51c-bbcf-4d5a-be42-ce850f39ca96/tsta_a_1145531_f0011_oc.gif)
Figure 12. Color rendition of the transconductance in (N, B) plane – the tiny pattern agrees with the hierarchy for monolayer graphene given in Table (after [Citation39]).
![Figure 12. Color rendition of the transconductance in (N, B) plane – the tiny pattern agrees with the hierarchy for monolayer graphene given in Table 1 (after [Citation39]).](/cms/asset/0dac98ab-20df-4080-9a6f-a929ad15fa7c/tsta_a_1145531_f0012_oc.gif)
Figure 13. Observation of FQHE at T = 0.25 K in bilayer suspended graphene. Magneto-resistance Rxx (blue curve) and Rxy (black curve) at the lateral voltage –27 V (after [Citation1]). Red symbols represent ratios from the hierarchy given in Table .
![Figure 13. Observation of FQHE at T = 0.25 K in bilayer suspended graphene. Magneto-resistance Rxx (blue curve) and Rxy (black curve) at the lateral voltage –27 V (after [Citation1]). Red symbols represent ratios from the hierarchy given in Table 2.](/cms/asset/f6a29720-c33a-4eb7-bbfd-019681ae4a5e/tsta_a_1145531_f0013_oc.gif)