Figures & data
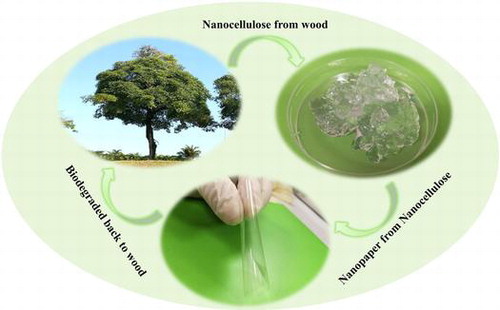
Figure 1. (a) Schematic of hierarchical structure of wood fibers [Citation2]. Reproduced with the permission of [Citation2]. Copyright 2013 The Royal Society of Chemistry. (b) Chemical structure of cellulose [Citation12]. Reproduced with the permission of [Citation12]. Copyright 2010 American Chemical Society.
![Figure 1. (a) Schematic of hierarchical structure of wood fibers [Citation2]. Reproduced with the permission of [Citation2]. Copyright 2013 The Royal Society of Chemistry. (b) Chemical structure of cellulose [Citation12]. Reproduced with the permission of [Citation12]. Copyright 2010 American Chemical Society.](/cms/asset/977b7c4b-50ab-4faa-844c-d9c9967029b0/tsta_a_1364976_f0001_oc.gif)
Figure 2. Various transparent papers made of different cellulose materials. (a) Transparent nanocomposite paper prepared by using NCCs and glycerol [Citation31]. Reproduced with the permission of [Citation31]. Copyright 2013 Nature Publishing. (b) Transparent nanopaper prepared by using NCCs [Citation32]. Reproduced with the permission of [Citation32]. Copyright 2012 American Chemical Society. (c) Transparent nanopaper obtained from NFCs by vacuum filtration [Citation41]. Reproduced with the permission of [Citation41]. Copyright 2015 Wiley-VCH. (d) Transparent nanopaper made of TEMPO-oxidized NFCs by vacuum filtration [Citation42]. Reproduced with the permission of [Citation42]. Copyright 2016 Wiley-VCH. (e) Transparent nanopaper made of BC sheet [Citation22]. Reproduced with the permission of [Citation22]. Copyright 2016 Springer Publishing. (f) Transparent nanocomposite paper prepared by using BC sheet with acrylic resin [Citation23]. Reproduced with the permission of [Citation23]. Copyright 2005 Wiley-VCH.
![Figure 2. Various transparent papers made of different cellulose materials. (a) Transparent nanocomposite paper prepared by using NCCs and glycerol [Citation31]. Reproduced with the permission of [Citation31]. Copyright 2013 Nature Publishing. (b) Transparent nanopaper prepared by using NCCs [Citation32]. Reproduced with the permission of [Citation32]. Copyright 2012 American Chemical Society. (c) Transparent nanopaper obtained from NFCs by vacuum filtration [Citation41]. Reproduced with the permission of [Citation41]. Copyright 2015 Wiley-VCH. (d) Transparent nanopaper made of TEMPO-oxidized NFCs by vacuum filtration [Citation42]. Reproduced with the permission of [Citation42]. Copyright 2016 Wiley-VCH. (e) Transparent nanopaper made of BC sheet [Citation22]. Reproduced with the permission of [Citation22]. Copyright 2016 Springer Publishing. (f) Transparent nanocomposite paper prepared by using BC sheet with acrylic resin [Citation23]. Reproduced with the permission of [Citation23]. Copyright 2005 Wiley-VCH.](/cms/asset/c81fb7c5-e8fa-4210-a27b-85f54d62bbd4/tsta_a_1364976_f0002_oc.gif)
Figure 3. Schematics for the folding procedure. (b) Sheet resistance versus the number of ±180° folding cycles. Images of the EC nanopaper in the (c) bleached state and (d) colored state. (e) Switching rate test [Citation41]. Reproduced with the permission of [Citation41]. Copyright 2015 Wiley-VCH.
![Figure 3. Schematics for the folding procedure. (b) Sheet resistance versus the number of ±180° folding cycles. Images of the EC nanopaper in the (c) bleached state and (d) colored state. (e) Switching rate test [Citation41]. Reproduced with the permission of [Citation41]. Copyright 2015 Wiley-VCH.](/cms/asset/8dea541b-55c9-4c83-a0c6-dcf2fc2c6cfd/tsta_a_1364976_f0003_oc.gif)
Figure 4. (a) Sheet resistance versus the number of ±180° folding cycles. Images of the foldability test of the SWCNT@Ag nanopaper electrode (b) before and (c) after crumpling. (d) Schematic of the solid state EC device. (e) Demonstration of solid state EC device mounted on a bent wrist [Citation42]. Reproduced with the permission of [Citation42]. Copyright 2016 Wiley-VCH.
![Figure 4. (a) Sheet resistance versus the number of ±180° folding cycles. Images of the foldability test of the SWCNT@Ag nanopaper electrode (b) before and (c) after crumpling. (d) Schematic of the solid state EC device. (e) Demonstration of solid state EC device mounted on a bent wrist [Citation42]. Reproduced with the permission of [Citation42]. Copyright 2016 Wiley-VCH.](/cms/asset/37decbd6-0857-4384-a52d-fdd89e194a06/tsta_a_1364976_f0004_oc.gif)
Figure 5. (a) Schematic structure of a four-wire resistive touch screen with CNT and transparent nanopaper electrode. (b) The word ‘paper’ was demonstrated in an assembled paper touch screen [Citation4]. Reproduced with the permission of [Citation4]. Copyright 2013 The Royal Society of Chemistry. (c) Schematic of super clear paper-based multipoint capacitive touch screen. (d) Optical of the whole touch screen device. (e) Measurement of linearity of paper-based touch screen [Citation62]. Reproduced with the permission of [Citation62]. Copyright 2016 American Chemical Society.
![Figure 5. (a) Schematic structure of a four-wire resistive touch screen with CNT and transparent nanopaper electrode. (b) The word ‘paper’ was demonstrated in an assembled paper touch screen [Citation4]. Reproduced with the permission of [Citation4]. Copyright 2013 The Royal Society of Chemistry. (c) Schematic of super clear paper-based multipoint capacitive touch screen. (d) Optical of the whole touch screen device. (e) Measurement of linearity of paper-based touch screen [Citation62]. Reproduced with the permission of [Citation62]. Copyright 2016 American Chemical Society.](/cms/asset/769b50e0-e8de-43aa-aa98-a6b020ebde4c/tsta_a_1364976_f0005_oc.gif)
Figure 6. (a) Schematic structure of the organic solar cell on NCC substrates. (b) Image of an assembled solar cell. (c) I-V curve of the solar cell on NCC substrates [Citation31]. Reproduced with the permission of [Citation31]. Copyright 2013 Nature Publishing. (d) Digital image of the transparent nanopaper with ultra-high haze prepared from TEMPO-oxidized wood fibers. (e) Structure of the solar cell device with ultra-high haze nanopaper attached on the opposite glass side. (f) I-V curves of the solar cell with/without transparent nanopaper made of wood fibers [Citation65]. Reproduced with the permission of [Citation65]. Copyright 2014 American Chemical Society.
![Figure 6. (a) Schematic structure of the organic solar cell on NCC substrates. (b) Image of an assembled solar cell. (c) I-V curve of the solar cell on NCC substrates [Citation31]. Reproduced with the permission of [Citation31]. Copyright 2013 Nature Publishing. (d) Digital image of the transparent nanopaper with ultra-high haze prepared from TEMPO-oxidized wood fibers. (e) Structure of the solar cell device with ultra-high haze nanopaper attached on the opposite glass side. (f) I-V curves of the solar cell with/without transparent nanopaper made of wood fibers [Citation65]. Reproduced with the permission of [Citation65]. Copyright 2014 American Chemical Society.](/cms/asset/f23b5657-585e-489f-b40f-38381f9b78aa/tsta_a_1364976_f0006_oc.gif)
Figure 7. (a) Cross section of fabricated organic TFT on nanopaper substrate. Photographs of 20-μm-thick transparent nanopaper-based organic TFT array in (b) bending and (c) folding states [Citation68]. Reproduced with the permission of [Citation68]. Copyright 2014 Wiley-VCH. (d) Schematic and cross section of MoS2 phototransistor with flexible nanopaper as substrate. (e) Optical transmittance of nanopaper and the sealed phototransistor. (f) Image of an array of phototransistor [Citation69]. Reproduced with the permission of [Citation69]. Copyright 2016 The Royal Society of Chemistry.
![Figure 7. (a) Cross section of fabricated organic TFT on nanopaper substrate. Photographs of 20-μm-thick transparent nanopaper-based organic TFT array in (b) bending and (c) folding states [Citation68]. Reproduced with the permission of [Citation68]. Copyright 2014 Wiley-VCH. (d) Schematic and cross section of MoS2 phototransistor with flexible nanopaper as substrate. (e) Optical transmittance of nanopaper and the sealed phototransistor. (f) Image of an array of phototransistor [Citation69]. Reproduced with the permission of [Citation69]. Copyright 2016 The Royal Society of Chemistry.](/cms/asset/4e9bcafe-9d22-4ecb-9308-f62b76f7c2fe/tsta_a_1364976_f0007_oc.gif)
Figure 8. (a) Luminescence of an OLED fabricated on transparent BNC nanocomposite paper [Citation50]. Reproduced with the permission of [Citation50]. Copyright 2008 Wiley-VCH. (b) Image of an OLED deposited on pure nanopaper in operation. (c) I-V curve of flexible OLED based on transparent nanopaper in flat and bent states, respectively. The inset is the schematic of a nanopaper OLED device [Citation71]. Reproduced with the permission of [Citation71]. Copyright 2013 The Royal Society of Chemistry. (d) Schematic of the light trapping effect of nanocellulose translucent film attached on the Si wafer. (e) Current density and voltage curves with and without nanocellulose film. (f) The effect of film on illuminous efficacy-voltage curves [Citation73]. Reproduced with the permission of [Citation73]. Copyright 2015 American Chemical Society.
![Figure 8. (a) Luminescence of an OLED fabricated on transparent BNC nanocomposite paper [Citation50]. Reproduced with the permission of [Citation50]. Copyright 2008 Wiley-VCH. (b) Image of an OLED deposited on pure nanopaper in operation. (c) I-V curve of flexible OLED based on transparent nanopaper in flat and bent states, respectively. The inset is the schematic of a nanopaper OLED device [Citation71]. Reproduced with the permission of [Citation71]. Copyright 2013 The Royal Society of Chemistry. (d) Schematic of the light trapping effect of nanocellulose translucent film attached on the Si wafer. (e) Current density and voltage curves with and without nanocellulose film. (f) The effect of film on illuminous efficacy-voltage curves [Citation73]. Reproduced with the permission of [Citation73]. Copyright 2015 American Chemical Society.](/cms/asset/4fa706fe-143a-44c8-9b4a-beb306274061/tsta_a_1364976_f0008_oc.gif)
Figure 9. (a) Schematic illustrating the transparent nanopaper-based actuator for art anti-theft and mapping anti-forgery applications [Citation75]. Reproduced with the permission of [Citation75]. Copyright 2015 American Chemical Society. (b) Digital images of the degradation process for transparent nanopaper. (c) The weight loss of the nanogenerator device in soil. (d) The tensile strength change of PLA in soil [Citation76]. Reproduced with the permission of [Citation76]. Copyright 2016 American Chemical Society.
![Figure 9. (a) Schematic illustrating the transparent nanopaper-based actuator for art anti-theft and mapping anti-forgery applications [Citation75]. Reproduced with the permission of [Citation75]. Copyright 2015 American Chemical Society. (b) Digital images of the degradation process for transparent nanopaper. (c) The weight loss of the nanogenerator device in soil. (d) The tensile strength change of PLA in soil [Citation76]. Reproduced with the permission of [Citation76]. Copyright 2016 American Chemical Society.](/cms/asset/276d5390-4292-46ee-995a-03f8866707e2/tsta_a_1364976_f0009_oc.gif)
Figure 10. (a) The V-shaped antenna by screen-printing silver nanowire ink on transparent nanopaper. (b) Scanning electron microscopy image of the silver nanowire on nanopaper. (c) Return loss of nanopaper antennae under folded and unfolded states [Citation77]. Reproduced with the permission of [Citation77]. Copyright 2013 The Royal Society of Chemistry. (d) The mechanism of the shape stability improves with glutaraldehyde treatment on transparent nanopaper. (e) Schematic of the gravure printing proofer. (f) Digital photo of four RFID antennas on cross-linked nanopaper [Citation79]. Reproduced with the permission of [Citation79]. Copyright 2014 The Royal Society of Chemistry.
![Figure 10. (a) The V-shaped antenna by screen-printing silver nanowire ink on transparent nanopaper. (b) Scanning electron microscopy image of the silver nanowire on nanopaper. (c) Return loss of nanopaper antennae under folded and unfolded states [Citation77]. Reproduced with the permission of [Citation77]. Copyright 2013 The Royal Society of Chemistry. (d) The mechanism of the shape stability improves with glutaraldehyde treatment on transparent nanopaper. (e) Schematic of the gravure printing proofer. (f) Digital photo of four RFID antennas on cross-linked nanopaper [Citation79]. Reproduced with the permission of [Citation79]. Copyright 2014 The Royal Society of Chemistry.](/cms/asset/2c7d3a09-2ab7-4b86-96c8-de7bffec2b28/tsta_a_1364976_f0010_oc.gif)