Figures & data
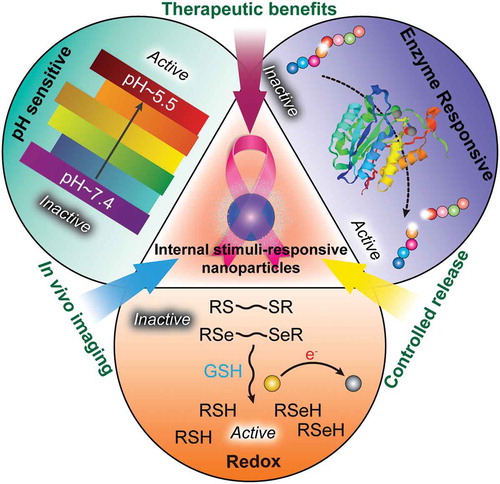
Figure 1. Development of functional biomimetic nanoparticles for precision drug delivery applications in cancer treatment.
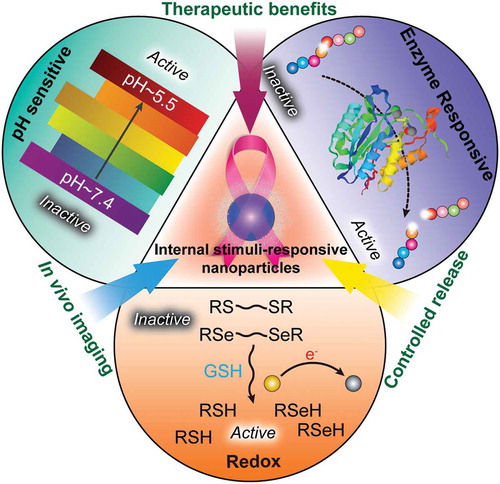
Figure 2. (a) Schematics of pH-modulation of fluorescence emission changes of PMAA-b-P(PEGMA-co-BODIPY) and its self-assembled multifunctional micelles. (b) Illustration of pH-sensitive drug-loaded micelles based on the PMAA-b-P(PEGMA-co-BODIPY) block copolymer for the intracellular release of DOX triggered by the acidic microenvironment. Reproduced with permission from Ref. [Citation54]. Copyright 2015 Royal Society of Chemistry.
![Figure 2. (a) Schematics of pH-modulation of fluorescence emission changes of PMAA-b-P(PEGMA-co-BODIPY) and its self-assembled multifunctional micelles. (b) Illustration of pH-sensitive drug-loaded micelles based on the PMAA-b-P(PEGMA-co-BODIPY) block copolymer for the intracellular release of DOX triggered by the acidic microenvironment. Reproduced with permission from Ref. [Citation54]. Copyright 2015 Royal Society of Chemistry.](/cms/asset/ca34e717-e29d-4086-b6ba-3dc1a95834e1/tsta_a_1528850_f0002_oc.jpg)
Table 1. Applications of pH-sensitive liposomes for anticancer drug delivery.
Figure 3. Design of MGlu-Dex-modified liposomes for induction of antigen-specific immunity. MGlu-Dex-modified liposome is taken up by DC via endocytosis and trapped in endosome. CTL stands for cytotoxic T lymphocyte. Its weakly acidic environment triggers destabilization of the liposome, which induces release of antigen molecules in endosome and their transfer to cytosol via fusion with endosome. Reproduced with permission from Ref. [Citation71]. Copyright 2013 Elsevier Ltd.
![Figure 3. Design of MGlu-Dex-modified liposomes for induction of antigen-specific immunity. MGlu-Dex-modified liposome is taken up by DC via endocytosis and trapped in endosome. CTL stands for cytotoxic T lymphocyte. Its weakly acidic environment triggers destabilization of the liposome, which induces release of antigen molecules in endosome and their transfer to cytosol via fusion with endosome. Reproduced with permission from Ref. [Citation71]. Copyright 2013 Elsevier Ltd.](/cms/asset/9899b0d9-6ae2-42a8-879c-78adfa7be8de/tsta_a_1528850_f0003_oc.jpg)
Figure 4. Schematic illustration of antigen delivery routes for free peptide and peptide-loaded pH-sensitive polymer-modified liposomes. Reproduced with permission from Ref. [Citation87]. Copyright 2016 Creative Commons.
![Figure 4. Schematic illustration of antigen delivery routes for free peptide and peptide-loaded pH-sensitive polymer-modified liposomes. Reproduced with permission from Ref. [Citation87]. Copyright 2016 Creative Commons.](/cms/asset/1433cb5e-3c21-4c53-87c3-e12685acef69/tsta_a_1528850_f0004_oc.jpg)
Figure 5. Schematic illustration of intracellular drug release from ZnO@MSN. Reproduced with permission from Ref. [Citation95]. Copyright 2015 American Chemical Society.
![Figure 5. Schematic illustration of intracellular drug release from ZnO@MSN. Reproduced with permission from Ref. [Citation95]. Copyright 2015 American Chemical Society.](/cms/asset/11310b70-fd45-4f50-a190-b9d5a50f1592/tsta_a_1528850_f0005_oc.jpg)
Figure 6. Schematic illustration of the design, construction, and application of light-up CMINs for monitoring of miR-34a replacement efficacy and accurate imaging-guided cancer therapy. ICG and miR-34a were simultaneously co-embedded in the nanocomplexes and subsequently stabilized by intermolecular cross-linking referred to as CMINs, which could better protect the embedded miR-34a and ICG and insure their release especially when dissociation of nanocomplexes happens. (A) Chemical structures of the components and preparation procedures of CMINs. (B) Schematic of the accumulation of CMINs in tumor sites via EPR effect (passive targeting). (C) Schematic of GSH-mediated dissociation of CMINs and the release of ICG and miR-34a upon intracellular uptake of nanocomplexes. Reproduced with permission from Ref. [Citation109]. Copyright 2018 Wiley.
![Figure 6. Schematic illustration of the design, construction, and application of light-up CMINs for monitoring of miR-34a replacement efficacy and accurate imaging-guided cancer therapy. ICG and miR-34a were simultaneously co-embedded in the nanocomplexes and subsequently stabilized by intermolecular cross-linking referred to as CMINs, which could better protect the embedded miR-34a and ICG and insure their release especially when dissociation of nanocomplexes happens. (A) Chemical structures of the components and preparation procedures of CMINs. (B) Schematic of the accumulation of CMINs in tumor sites via EPR effect (passive targeting). (C) Schematic of GSH-mediated dissociation of CMINs and the release of ICG and miR-34a upon intracellular uptake of nanocomplexes. Reproduced with permission from Ref. [Citation109]. Copyright 2018 Wiley.](/cms/asset/c44cb23b-372c-4255-9ced-b237d31fe2f3/tsta_a_1528850_f0006_oc.jpg)
Figure 7. (a, b) Fabrication of redox-trigged HMSNs by using a disulfide bond as the intermediate linker. (c) Illustration of the intracellular redox-trigged HMSNs for targeted tumor therapy in vitro and in vivo. Reproduced with permission from Ref. [Citation110]. Copyright Ivyspring International Publisher.
![Figure 7. (a, b) Fabrication of redox-trigged HMSNs by using a disulfide bond as the intermediate linker. (c) Illustration of the intracellular redox-trigged HMSNs for targeted tumor therapy in vitro and in vivo. Reproduced with permission from Ref. [Citation110]. Copyright Ivyspring International Publisher.](/cms/asset/d1fcc60c-7374-408a-99f7-9fee33d87b1c/tsta_a_1528850_f0007_oc.jpg)
Figure 8. Tissues containing healthy (pink) and tumor (gray) cells can be treated with various nanomaterials, such as (from left to right) liposomes, protein-conjugates, polymeric nanoparticles, hydrogels, dendrimers, and inorganic metal nanoparticles, to deliver imaging agents or anticancer drugs with improved selectivity to tumor cells by incorporation of protease-responsiveness into the design of nanomaterials. Reproduced with permission from Ref. [Citation128]. Copyright 2017 American Chemical Society.
![Figure 8. Tissues containing healthy (pink) and tumor (gray) cells can be treated with various nanomaterials, such as (from left to right) liposomes, protein-conjugates, polymeric nanoparticles, hydrogels, dendrimers, and inorganic metal nanoparticles, to deliver imaging agents or anticancer drugs with improved selectivity to tumor cells by incorporation of protease-responsiveness into the design of nanomaterials. Reproduced with permission from Ref. [Citation128]. Copyright 2017 American Chemical Society.](/cms/asset/4fe4e034-1fe3-4c90-b7e4-cc18ad464f55/tsta_a_1528850_f0008_oc.jpg)
Figure 9. Schematic representation of nanovesicles incorporating MMP-9 substrate lipopeptides and reduction-sensitive POPE-SS-PEG which render the nanovesicles responsive to extracellular, elevated levels of MMP-9 and GSH. Reproduced with permission from Ref. [Citation130]. Copyright 2014 American Chemical Society.
![Figure 9. Schematic representation of nanovesicles incorporating MMP-9 substrate lipopeptides and reduction-sensitive POPE-SS-PEG which render the nanovesicles responsive to extracellular, elevated levels of MMP-9 and GSH. Reproduced with permission from Ref. [Citation130]. Copyright 2014 American Chemical Society.](/cms/asset/ed6aa65a-e10d-418d-91e6-a807dbc370fd/tsta_a_1528850_f0009_oc.jpg)
Figure 10. In vivo biodistribution and in vitro fluorescence images of major organs and tumors. (A) In vivo time-dependent whole body fluorescence imaging of A549 tumor-bearing mice after intravenous injection of probes. (B) Quantification of average fluorescence signals in the tumor site of panel (A). (C) In vitro fluorescence images of major organs and tumors of mice after intravenous injection of probes over a period of 36 h. (D) Quantification of average fluorescence signals of panel C. Reproduced with permission from Ref. [Citation131]. Copyright 2016 American Chemical Society.
![Figure 10. In vivo biodistribution and in vitro fluorescence images of major organs and tumors. (A) In vivo time-dependent whole body fluorescence imaging of A549 tumor-bearing mice after intravenous injection of probes. (B) Quantification of average fluorescence signals in the tumor site of panel (A). (C) In vitro fluorescence images of major organs and tumors of mice after intravenous injection of probes over a period of 36 h. (D) Quantification of average fluorescence signals of panel C. Reproduced with permission from Ref. [Citation131]. Copyright 2016 American Chemical Society.](/cms/asset/585ea04e-c05d-44b9-87fb-a9f6f12501e4/tsta_a_1528850_f0010_oc.jpg)