Figures & data
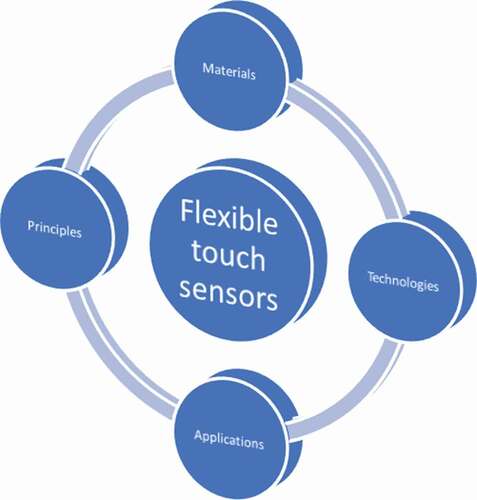
Figure 1. Flexible touch sensors, consisting of Principles, Materials, Technologies, and Applications in e-skins. Reproduced with permission from Ref [Citation6]. copyright 2019 Elsevier B.V., e-textiles. Adapted with permission from Ref [Citation11]. copyright 2018 Springer Nature, e-healthcare. Adapted with permission from Ref [Citation7]. copyright 2017 American Chemical Society, e-control. Adapted with permission from Ref [Citation5]. copyright 2020 IEEE
![Figure 1. Flexible touch sensors, consisting of Principles, Materials, Technologies, and Applications in e-skins. Reproduced with permission from Ref [Citation6]. copyright 2019 Elsevier B.V., e-textiles. Adapted with permission from Ref [Citation11]. copyright 2018 Springer Nature, e-healthcare. Adapted with permission from Ref [Citation7]. copyright 2017 American Chemical Society, e-control. Adapted with permission from Ref [Citation5]. copyright 2020 IEEE](/cms/asset/5e0bb8b1-23af-4e87-8750-b4d524906931/tsta_a_1862629_f0001_oc.jpg)
Table 1. Summary the electrical and mechanical performance of the different sensors and sensing technologies, where PDMS is polydimethylsiloxane, CNTs are carbon nanotubes, SWNTs are single-walled carbon nanotubes, ITO is indium tin oxide, CNFs are carbon nanofibers, PPy is polypyrrole, BCZT is (Ba0.85Ca0.15)(Ti0.90Zr0.10)O3, AgNWs are silver nanowires
Figure 2. Schematic illustrations: (a) The flexible electrode using spin coating with electron-induced perpendicular graphene (EIPG), anodic aluminum oxide (AAO), and polydimethylsiloxane (PDMS). Reproduced with permission from Ref [Citation4]. copyright 2020 Springer Nature. (b) The inkjet-printing controllable process for the evaporation-driven convective particle self-assembly at the contact line. Adapted with permission from Ref [Citation73]. copyright 2020 Wiley-VCH. (c) The electrospinning and electrospraying processes of the AgNF-AgNW hybrid electrode. Adapted with permission from Ref [Citation53]. copyright 2018 Springer Nature
![Figure 2. Schematic illustrations: (a) The flexible electrode using spin coating with electron-induced perpendicular graphene (EIPG), anodic aluminum oxide (AAO), and polydimethylsiloxane (PDMS). Reproduced with permission from Ref [Citation4]. copyright 2020 Springer Nature. (b) The inkjet-printing controllable process for the evaporation-driven convective particle self-assembly at the contact line. Adapted with permission from Ref [Citation73]. copyright 2020 Wiley-VCH. (c) The electrospinning and electrospraying processes of the AgNF-AgNW hybrid electrode. Adapted with permission from Ref [Citation53]. copyright 2018 Springer Nature](/cms/asset/64056936-1114-4581-b2e4-7e5daae99b32/tsta_a_1862629_f0002_oc.jpg)
Figure 3. (a) Three-dimensional fingertip-shaped artificial skin device by 3D printing, which can sense exact touch location and heal mechanical damage spontaneously. Reproduced with permission from Ref [Citation80]. copyright 2019 American Chemical Society. (b) Schematic illustration of the e‐skin consisting of two electrodes and the natural material (flower and leaf) as the dielectric layer in between. Besides, the graphs show the capacitance change and the sensitivity when applied pressure on three types of e-skins based critical point dried rose petal, rose leaf, and acacia leaf. Adapted with permission from Ref [Citation81]. copyright 2018 Wiley-VCH. (c) Soft-touch textile sensors in high-voltage TASER test to short out the electrical shock. Adapted with permission from Ref [Citation86]. copyright 2020 American Chemical Society. (d) Omniphobic triboelectric nanogenerators (RF‐TENGs) from e-textiles with the shape of a cat powering two LEDs embroidered as eyes (when touch). Adapted with permission from Ref [Citation88]. copyright 2019 Wiley-VCH
![Figure 3. (a) Three-dimensional fingertip-shaped artificial skin device by 3D printing, which can sense exact touch location and heal mechanical damage spontaneously. Reproduced with permission from Ref [Citation80]. copyright 2019 American Chemical Society. (b) Schematic illustration of the e‐skin consisting of two electrodes and the natural material (flower and leaf) as the dielectric layer in between. Besides, the graphs show the capacitance change and the sensitivity when applied pressure on three types of e-skins based critical point dried rose petal, rose leaf, and acacia leaf. Adapted with permission from Ref [Citation81]. copyright 2018 Wiley-VCH. (c) Soft-touch textile sensors in high-voltage TASER test to short out the electrical shock. Adapted with permission from Ref [Citation86]. copyright 2020 American Chemical Society. (d) Omniphobic triboelectric nanogenerators (RF‐TENGs) from e-textiles with the shape of a cat powering two LEDs embroidered as eyes (when touch). Adapted with permission from Ref [Citation88]. copyright 2019 Wiley-VCH](/cms/asset/a01ada34-9f7f-446f-a9ce-e8bd94d105bd/tsta_a_1862629_f0003_oc.jpg)
Figure 4. a) The photoplethysmogram (PPG) sensor hybrids an organic phototransistor (OPT) with an inorganic light‐emitting diode for cardiovascular monitoring, consisting of the fabrication process, the real picture of the sensor, and the OPT-LED structure are directly laminated onto the finger. Reproduced with permission from Ref [Citation93]. copyright 2017 Wiley-VCH. b) The fingers of the hand are attached to the nanomesh film devices for tissue-temperature sensing and schematic illustration of remote monitoring of the sensing performance of the assembled film devices by wireless communication with a mobile phone. Adapted with permission from Ref [Citation94]. copyright 2019 Wiley-VCH
![Figure 4. a) The photoplethysmogram (PPG) sensor hybrids an organic phototransistor (OPT) with an inorganic light‐emitting diode for cardiovascular monitoring, consisting of the fabrication process, the real picture of the sensor, and the OPT-LED structure are directly laminated onto the finger. Reproduced with permission from Ref [Citation93]. copyright 2017 Wiley-VCH. b) The fingers of the hand are attached to the nanomesh film devices for tissue-temperature sensing and schematic illustration of remote monitoring of the sensing performance of the assembled film devices by wireless communication with a mobile phone. Adapted with permission from Ref [Citation94]. copyright 2019 Wiley-VCH](/cms/asset/f2c85c4d-e26c-463b-aaec-a95385188e4c/tsta_a_1862629_f0004_oc.jpg)
Figure 5. Application of the programmable touch operation platform based on the user-interactive e-skin (SUE-skin) for tracks recognition: (a) Optical photograph and four-electrodes schematic of the SUE-skin. (b) Light intensity and output voltage of the SUE-skin under different pressures and different prestress. (c) Touch operation demonstration for the audio controlling and the characters displaying. Adapted with permission from Ref [Citation95]. copyright 2018 Wiley-VCH
![Figure 5. Application of the programmable touch operation platform based on the user-interactive e-skin (SUE-skin) for tracks recognition: (a) Optical photograph and four-electrodes schematic of the SUE-skin. (b) Light intensity and output voltage of the SUE-skin under different pressures and different prestress. (c) Touch operation demonstration for the audio controlling and the characters displaying. Adapted with permission from Ref [Citation95]. copyright 2018 Wiley-VCH](/cms/asset/a36e5d34-1c03-4031-ac89-a6183d2ba798/tsta_a_1862629_f0005_oc.jpg)