Figures & data
Figure 1. Nanoarchitectonics strategy and its similarity to biological system: (A) outline of nanoarchitectonics approach; (B) common features between materials nanoarchitectonics and biological organization.
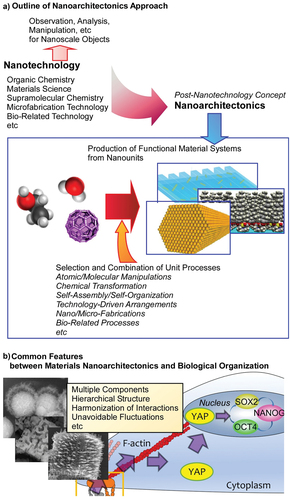
Figure 2. By incorporating pendant PEG to alginate that are either bound covalently, dynamically through Schiff base formation, or simply encapsulated (nonbinding), the irreversible plastic deformations can be controlled with respect to the Young’s modulus and stress relaxation.
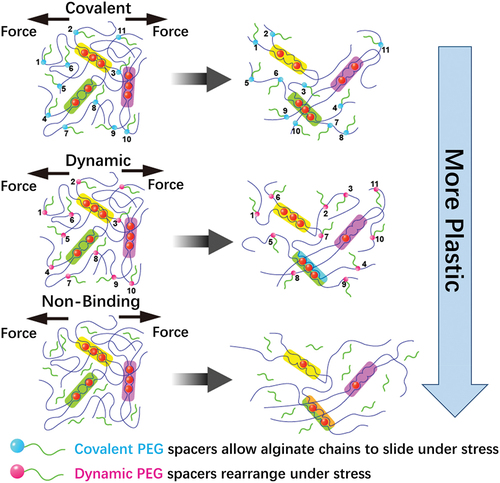
Figure 3. Schematic illustrations of cell culture at the liquid−liquid interfaces for control of cellular differentiation. (A) Myogenic differentiation was suppressed on fluid interface. (B) Interfacial culturing induced hMSCs differentiate into neural cells.
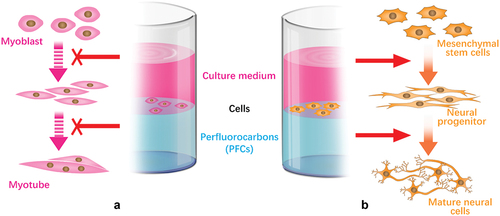
Figure 4. Different RGD nanospacings can alter hMSCs mechanosensing through differential coupling of FAK/Src/Rac1/myosin IIA/YAP/TAZ signalling pathways to support long-term changes in stem cell differentiation and state.
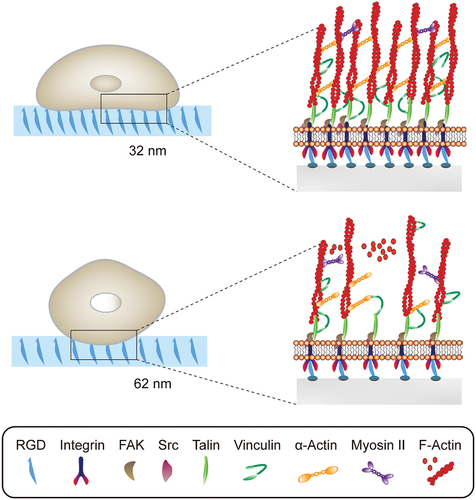
Figure 5. (A) SEM images of low-, medium-, and high- aligned FNWs nanopatterns. (B) Highly aligned fullerene nanowhisker nanopatterned scaffolds permit formation of mature focal adhesions and nucleus YAP translocation to promote hMSC multipotency retention.
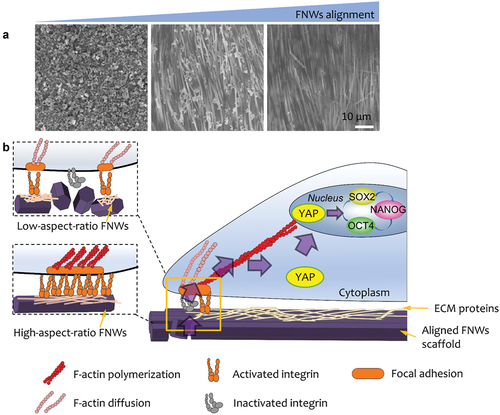
Figure 6. Study of the effect of Au nanorods (AuNRs)/polycaprolactone (PCL) thin films on macrophage phenotype. (A) Preparation and dynamic topography of shape memory AuNRs/PCL thin films. (B) Effects of topography of SMP on phenotypic changes of macrophages in rats. NIR triggered the transformation of SMP surface from flat to micro groove, which promoted the macrophage phenotype from iNOS+iL-10lo MΦ (circular) to Arg+iL-10hi MΦ (elongated) polarization. (C) SEM images of dynamic topography of SMP: primary micro-groove film, secondary flat film after compression and cooling, and NIR-triggered recovered micro-groove film. Scale bars are 50 μm [Citation148]. Copyright 2019 American Chemical Society.
![Figure 6. Study of the effect of Au nanorods (AuNRs)/polycaprolactone (PCL) thin films on macrophage phenotype. (A) Preparation and dynamic topography of shape memory AuNRs/PCL thin films. (B) Effects of topography of SMP on phenotypic changes of macrophages in rats. NIR triggered the transformation of SMP surface from flat to micro groove, which promoted the macrophage phenotype from iNOS+iL-10lo MΦ (circular) to Arg+iL-10hi MΦ (elongated) polarization. (C) SEM images of dynamic topography of SMP: primary micro-groove film, secondary flat film after compression and cooling, and NIR-triggered recovered micro-groove film. Scale bars are 50 μm [Citation148]. Copyright 2019 American Chemical Society.](/cms/asset/edcb3c1c-9e34-4910-b95d-9b348af65969/tsta_a_2082260_f0006_oc.jpg)
Figure 7. Preparation of EXO-PEF scaffold and its potential mechanism. (A) MSC exosomes were immobilized with PEI-modified electrospun fibres (PEFs) scaffolds by electrostatic interaction. (B) Potential immunomodulatory mechanism of EXO-PEF scaffold promoting tissue repair and regeneration: (i) recruitment of immune cells, (ii) training and promoting phenotypic transformation of immune cells, (iii) secretion of anti-inflammatory factors, and (iv) regulation of distant immune organs. (C) EXO-PEF cultured bone marrow-derived macrophages in vitro with lipopolysaccharides (LPS) simulation [Citation156]. Copyright 2021 AAAS.
![Figure 7. Preparation of EXO-PEF scaffold and its potential mechanism. (A) MSC exosomes were immobilized with PEI-modified electrospun fibres (PEFs) scaffolds by electrostatic interaction. (B) Potential immunomodulatory mechanism of EXO-PEF scaffold promoting tissue repair and regeneration: (i) recruitment of immune cells, (ii) training and promoting phenotypic transformation of immune cells, (iii) secretion of anti-inflammatory factors, and (iv) regulation of distant immune organs. (C) EXO-PEF cultured bone marrow-derived macrophages in vitro with lipopolysaccharides (LPS) simulation [Citation156]. Copyright 2021 AAAS.](/cms/asset/fceb5002-d9d1-4ab6-a2a7-db985d92d891/tsta_a_2082260_f0007_oc.jpg)
Figure 8. Schematic of MAP hydrogel preparation process and its effect on wound repair. (A) Diagrams of the preparation process of hydrogel microspheres. (B) Effect of L-MAP or D-MAP hydrogel applied to wound-healing model. Compared with L-MAP, D-MAP hydrogel can recruit immune cells and activate adaptive immunity, which further promotes tissue remodelling and regeneration and accelerates the in vivo degradation of D-MAP.
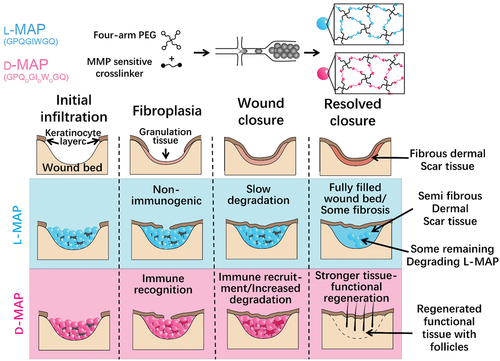
Figure 9. The effect of matrix stiffness on the immunomodulation of MSC. (A) Schematic of the effect of matrix stiffness on the immunomodulation of MSC. Under inflammatory conditions, ECM stiffness affects (1) the activation of MSC by inflammatory factor TNFα, and stimulates MSC to produce paracrine factors, thus affecting (3) the recruitment and (4) transport of monocytes. In bone marrow, the ECM near the endothelial cell region is softer (Young’s modulus E = 0.3–2 kPa), while the ECM near osteoblast region is stiffer (E = 30–100 kPa). (B) Mechanism of matrix stiffness regulating TNFα binding to cell surface. The soft matrix mediates the redistribution of actin polymerizations, and TNFα stimulates lipid rafts to promote TNFR 1 aggregation, thereby enhancing downstream gene expression. However, on stiff matrix, actin polymerization was reduced, hindering the binding of TNFα to cell surface TNFR 1.
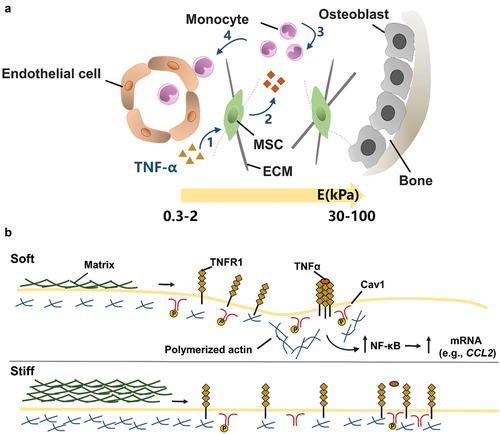
Figure 10. Inflammatory stimulation alters chemical and physio-mechanical signals in the cellular microenvironment, which influences MSC behaviours including: (1) the process of receiving soluble factors and (2) the paracrine effect of MSC. By designing gel coatings with defined chemomechanical cues, MSC regulation can be improved to (3) correct aberrantly ECM remodelling.
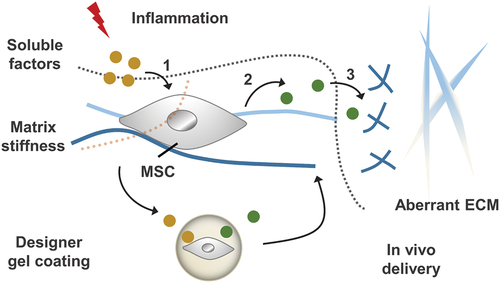
Figure 11. Developing renal organoids cultured within a perfusable millifluidic chip and subjected to controlled FSS exhibit enhanced vascularization and maturation.
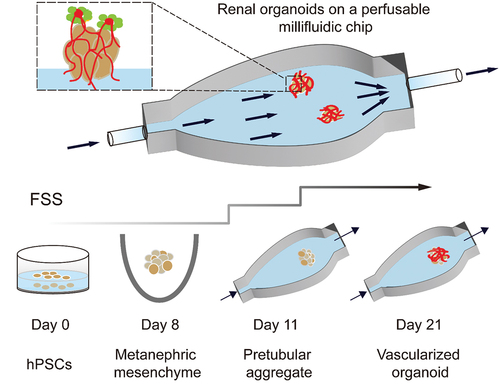