Figures & data
Figure 1. Mutations in the DMD gene. (a) In the healthy situation the dystrophin mRNA transcript encodes a fully functional dystrophin protein. (b) In Duchenne patients, mutations that disrupt the reading frame (e.g. a deletion of exon 52, left panel) or nonsense mutations (e.g. nonsense mutation in exon 42, right panel) lead to a premature truncation of protein translation and non-functional dystrophins. (c) In Becker patients mutations maintain the reading frame (a deletion of exon 51 and 52), allowing production of internally deleted, but partially functional proteins.
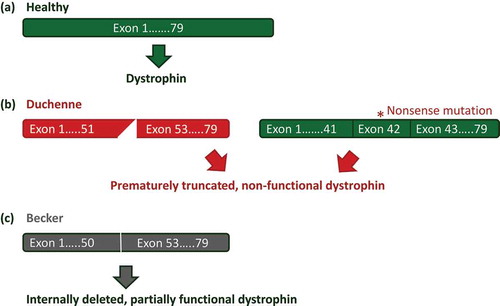
Figure 2. Antisense mediated exon skipping for various mutation types. (a) Antisense oligonucleotides (AONs) can hybridize to a target exon during the pre-mRNA splicing process. This will hide the target exon from the splicing machinery, and it will be spliced out with its flanking introns. For a deletion of exon 52, the skipping of exon 51 will restore the reading frame, to allow the production of a Becker-type dystrophin rather than a non-functional dystrophin. (b) Double exon skipping for a deletion mutation. For most deletions the reading frame can be corrected by skipping a single exon (see Figure 2). Some deletions however, such as a deletion of exon 46–50, required the skipping of both flanking exons to restore the reading frame. (c) Exon skipping for a splice site mutation. Most splice site mutations, will cause a single exon deletion on mRNA level (a 3ʹ splice site mutation in intron 44 results in exon 45 no longer being recognized by the splicing machinery in this example). Like with exon deletions, skipping additional exons (exon 46 in this example) can restore the reading frame. (d) Single exon skipping for small mutations. When small mutations are located within an in-frame exon, skipping this exon will bypass the mutation, while maintaining the reading frame. In this example, a 1 basepair insertion in exon 42 disrupts the reading frame. Skipping exon 42 bypasses the frame-disrupting mutation. (e) Double exon skipping for small mutations. When small mutations are located within out-of-frame exons, the mutation containing exon needs to be skipped as well as a flanking exon, to bypass the mutation without affecting the reading frame. In the example a nonsense mutation in exon 56 requires the skipping of both exon 56 and exon 57. (f) Exon skipping for single exon duplications. Single exon skipping for single exon duplications allows restoration of the normal transcript when either the original or duplicated exon is skipped (left panel). However, double exon skipping will lead to an out of frame transcript (right panel). (g) Exon skipping for cryptic splicing mutations. Deep intronic mutations can activate splice sites, leading to the inclusion of part of an intron into the mRNA. These cryptic exons often are out-of-frame and/or contain stop codons. Targeting the cryptic exon with an AON can restore the normal transcript.
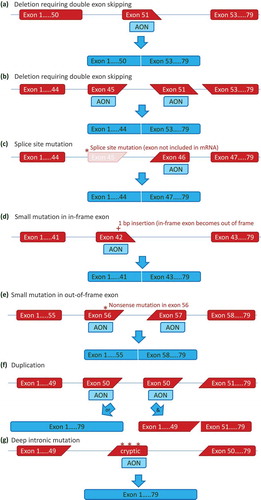
Figure 3. Dystrophin protein domains relative to the encoding exons (previously published [Citation28], used with permission from IOS press). Dystrophin function relies on the presence of at least one actin binding domain (encoded by exon 2–9 and exon 31–45) and the dystroglycan-binding domain (encoded by exon 64–70). Furthermore, the central rod domain (encoded by exon 10–63) does not tolerate deletions involving 36 or more exons.
![Figure 3. Dystrophin protein domains relative to the encoding exons (previously published [Citation28], used with permission from IOS press). Dystrophin function relies on the presence of at least one actin binding domain (encoded by exon 2–9 and exon 31–45) and the dystroglycan-binding domain (encoded by exon 64–70). Furthermore, the central rod domain (encoded by exon 10–63) does not tolerate deletions involving 36 or more exons.](/cms/asset/156132fe-ae15-48f4-9d15-77b93f3a3291/iebt_a_1271872_f0003_oc.jpg)
Figure 4. Genome editing approaches for DMD. (a) Deletion of a mutated or frame-disrupting exon. Using a pair of guide RNAs targeting the introns flanking an exon containing a mutation (the nonsense mutation in exon 23 from the mdx mouse is shown in this example), the exon can be removed on DNA level when the generated ends in intron 22 and intron 23 are linked by non homologous end joining. (b) Splice site mutations. A single guide RNA can be used to generate a double strand DNA break at a splice site. Since the non homologous end joining process is error prone, the repaired DNA will often involve a mutated splice site. Thus the exon containing the mutation is no longer recognized and skipped on RNA level, thus bypassing the mutation or restoring the reading frame. (c) Gene correction. In stem cells, double strand DNA breaks can activate homologous recombination (in addition to non homologous end joining). Homologous recombination requires a template with the correct information, that is provided with the guideRNA and CRISPR/Cas9. Homologous recombination between the homologous DNA at the borders of the DNA break and the template will result in a corrected DNA (exon 52 is restored in this example).
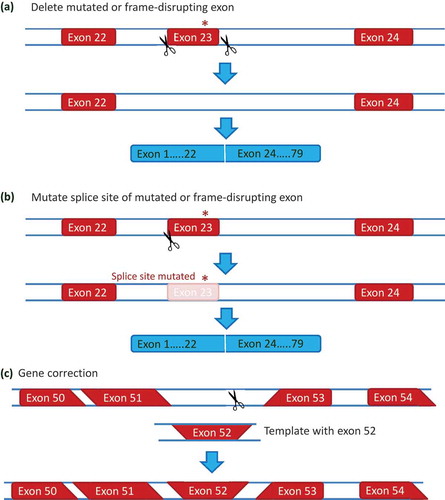