Figures & data

Figure 1. Structures of the studied compounds: (A) potential inhibitors with the covalent mode of action and (B) the non-covalent control analogues, derived from analogues with the non-covalent mechanism of action (C).
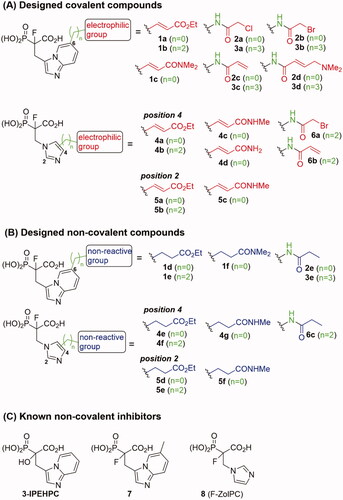
Table 2. Effects of Imidazo[1,2-a]pyridine Analogues of α-phosphonocarboxylates on HeLa Cell Viabilitya and Rab11A prenylationb
Table 3. Peptides identified in MS and intensity ratio of untreated vs treated with 2b inhibitor samples.
Scheme 1. Strategies used for the synthesis of α-phosphonocarboxylates 1. Reagents and conditions: (a) NaBH4, NiCl2·6H2O, MeOH; (b) for 12a,c and 14d,f (n = 0): N,N-dimethylacrylamide or ethyl acrylate, Pd(AcO)2, tri-o-tolylphosphine, DIPEA, propionitrile, microwave irradiation; for 12b and 14e (n = 2): (I) allyl alcohol, Pd(AcO)2, tri-o-tolylphosphine, DIPEA, propionitrile, microwave irradiation; (II) triethylphophonoacetate, NaH, THF; (c) NaH, NFSI, THF; (d) (I) bromotrimethylsilane, TEA, acetonitrile, (II) EtOH, (III) TFA.
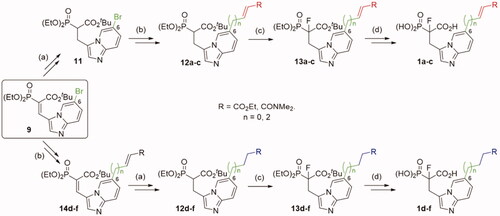
Scheme 2. Strategies used for the synthesis of α-phosphonocarboxylates 2–3. Reagents and conditions: (a) (I) 8, H2, Pd/C, (II) Boc2O, DCM, (III) NaBH4, NiCl2·6H2O, MeOH; (b) NaH, NFSI, THF; (c) for 2 b,c: (I) 3 M HCl/EtOH, (II) acyl halide, DCM; (d) (I) bromotrimethylsilane, TEA, acetonitrile, (II) EtOH, (III) TFA; (e) (I) 7, 2-allylisoindoline-1,3-dione, Pd(AcO)2, tri-o-tolylphosphine, DIPEA, propionitrile, microwave irradiation, (II) H2, Pd/C, (III) NaBH4, NiCl2·6H2O, MeOH; (f) (I) hydrazine hydrate, MeOH; (II) acyl halide or carboxylic acid with coupling agent in DCM or DMF; For details of synthesis of 2a,d, 3a see Schemes S7, S9.
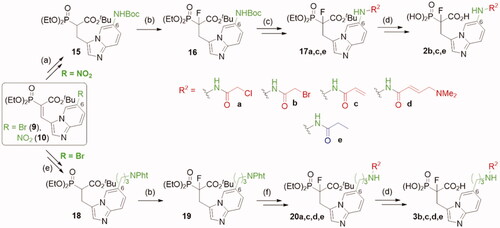
Scheme 3. Strategies used for the synthesis of α-phosphonocarboxylates 4–6. Reagents and conditions: (a) Scheme S10–14; (b) tert-butyl 2-(diethoxyphosphoryl)acrylate, NaH, THF, then NFSI, THF; (c) (I) bromotrimethylsilane, TEA, acetonitrile, (II) EtOH, (III) TFA; (d) (I) 23, phthalic anhydride, AcOH, (II) Ph3Cl, TEA, (III) hydrazine hydrate, MeOH, (IV) acyl halide, DCM, (V) TFA, DCM.
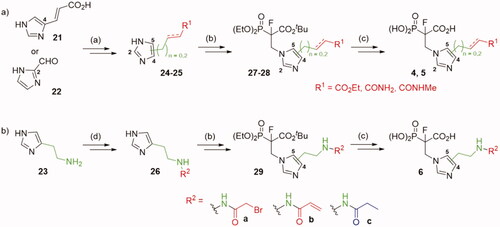
Table 1. Effect of exchanging electrophilic residue on half-Life Values t1/2 for reaction with GSH.
Figure 2. Effect of imidazo[1,2-a]pyridine (1–3; A) and imidazole (4–6; B) PC analogues on Rab11A prenylation in HeLa cells. Cells were treated for 48 h with PCs (100 µM). Rab11A and β-actin were detected in cytosolic fraction using Western blot.
![Figure 2. Effect of imidazo[1,2-a]pyridine (1–3; A) and imidazole (4–6; B) PC analogues on Rab11A prenylation in HeLa cells. Cells were treated for 48 h with PCs (100 µM). Rab11A and β-actin were detected in cytosolic fraction using Western blot.](/cms/asset/b6aa540e-d5d0-4c2f-8008-098f5e5ef030/ienz_a_2053525_f0002_b.jpg)
Figure 3. Effect of 6-substituted 3-IPEHPC analogue with the 3-ethoxy-3-oxopropyl group (1d), its version with an elongated carbon linker (n = 2; 1e), bromoacetamide analogue (2b) and its non-covalent counterpart (2e) on Rab11A and Rap1A/Rap1B prenylation in HeLa cells. Cells were treated for 48 h with the indicated concentrations of PCs (µM) or 10 µM lovastatin (Lov), acting as a positive control. Cytosolic fractions containing unprenylated proteins were Western blotted for Rab11A, Rap1A/Rap1B, and β-actin (A), and the bands were quantified by densitometry, normalised to β-actin, and presented as a percentage of controls (B). Data represented mean ± SEM from at least three independent experiments, *p ≤ 0.05, ****p ≤ 0.0001.
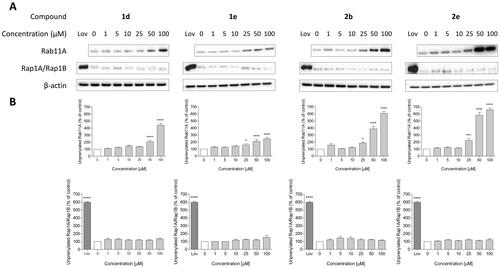
Figure 4. Effect of decomposition products of 2b and 3b on Rab11A prenylation in HeLa cells. Cells were treated for 48 h with 100 µM of freshly prepared 2b and 3b (-) and their solutions after incubation in PBS/D2O at 37 °C for 72 h (+). Cytosolic fractions containing unprenylated proteins were Western blotted for Rab11A and β-actin.
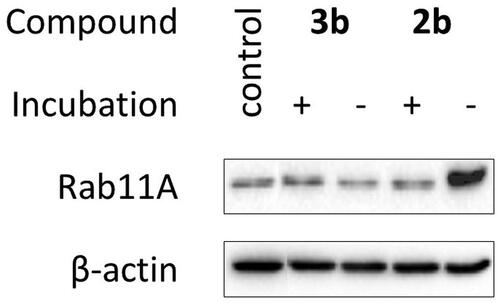
Figure 5. Possible covalent bond formation by compound 2b suggested by docking simulations using 4GTS structure. 2b adopts a similar binding mode as the non-covalent compound 717 (A). In not covalently bound state R144B stabilises 2b into the proximity of C196B (B) before the formation of the covalent interaction (C). The black dashed lines indicate interactions between the inhibitor and the protein. Black arrows indicate the formation of covalent bond. Used atom colours: C in protein amino acids, 7, 2b in unbound state, and 2b in bound state are grey, orange, light green, and light blue, respectively. O = red, N = blue, P = pink, S = yellow, F = dark green, Zn = golden, Br = magenta.
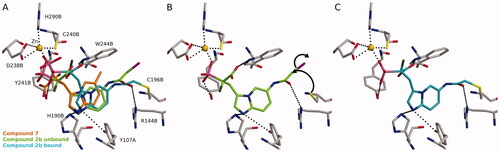