Figures & data
Figure 1. Methods for computational aeroacoustics (Wagner, Hüttl, and Sagaut Citation2007).
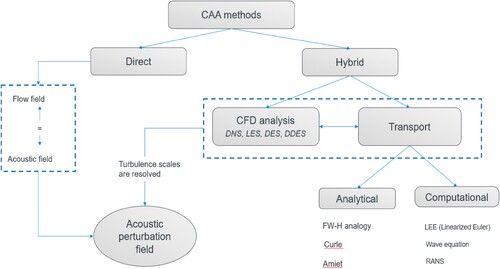
Figure 3. Interpretation of FW-H acoustic analogy in terms of source, observer regions and overlapping envelope surrounding the source and observer.
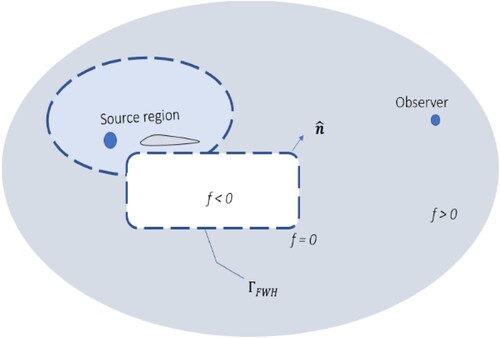
Table 1. General and wind turbine noise regulation standards and thresholds in different countries (Bhargava and Samala Citation2019a).
Figure 5. Resultant amplitude modulation of signal, f with a phase angle of 90° obtained using superimposing carrier signal (fc = 50 Hz, Ac = 1), and modulation signal, fm at modulation index, m = 0.9.
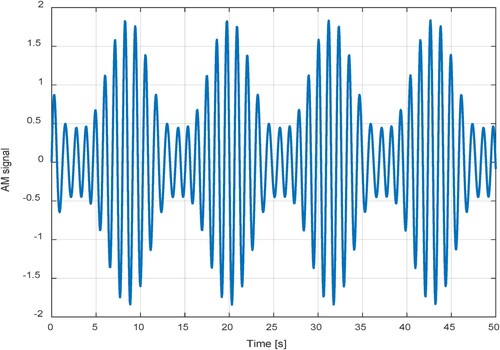
Figure 6. (a) Flow-induced noise sources from an aerofoil (Bhargava and Samala Citation2019b). (b) Illustration of wind turbine blade length (Bhargava and Samala Citation2019a).
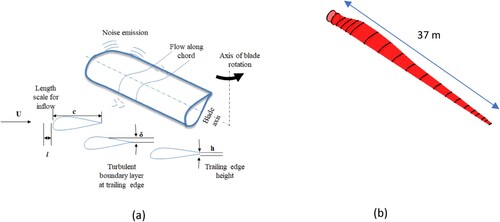
Figure 7. Illustration of sound pressure level varying with harmonic number, n, at wind speed of 13.5 m/s compared to experiment data measured downwind at distance of ∼100 m for (Δf = 0.25 Hz).
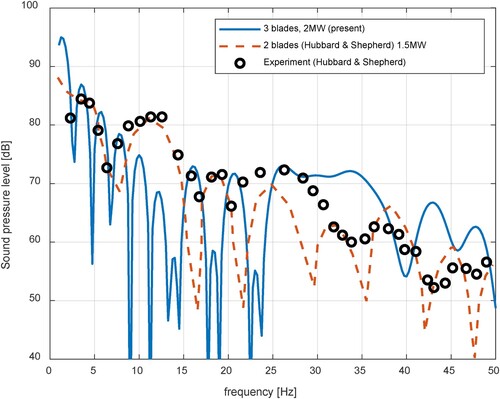
Figure 8. Experimental data for NACA 0012 aerofoil self-noise recorded in a wind tunnel for different test configurations (Sabat et al.).
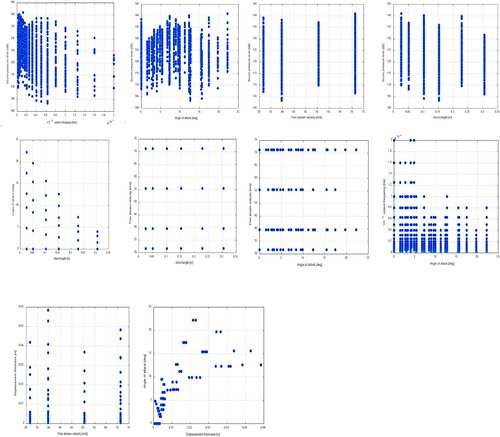
Table 2. Statistical descriptors for the aerofoil self-noise data (Viterna Citation1981).
Table 3. Summary of past research findings related to aerofoil self-noise prediction methods.
Figure 9. (a) One-third octave band frequency spectra of overall sound pressure level (with and without tip noise contribution) from NACA 0012 airfoil using BPM method having a chord length of 16cm and validated with 2D and 3D experiment wind tunnel data at U = 71.3 m/s and Angle of attack, α = 10° (Brooks, Pope, and Marcolini Citation1989). (b) Computed tip noise predicted from BPM model for square, round and sharp tip geometries for a 2MW wind turbine blade.
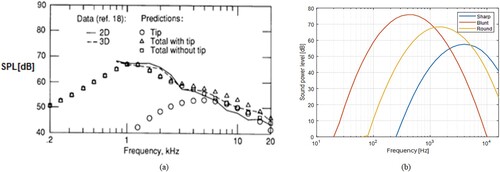
Figure 10. Self-noise spectra turbulent boundary layer trailing edge noise of NACA 0012 computed using BPM method at free stream velocity, U = 65 m/s (a) at 8° AOA for chord lengths of 1.5, 0.8 and 0.5 m (b) for chord length of 0.5 m at 2°, 4° and 8° angle of attack (Bhargava and Samala Citation2019b; Bhargava and Samala Citation2019a; Bhargava, Samala, and Anumula Citation2019).
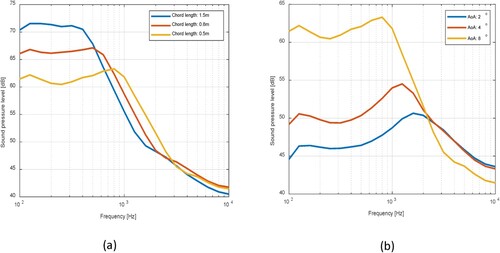
Figure 11. Comparison of (a) sound pressure (LAeq) and power (Lw) level difference with experiment data of Van den Berg (Citation2006) for different rotational speeds of three-bladed turbine (b) sound pressure (LAeq) predicted using 1x, 1.2x and 2x logarithmic velocity profile expressions for different wind speeds measured at 10 m height with experiment data of Van den Berg (Citation2006).
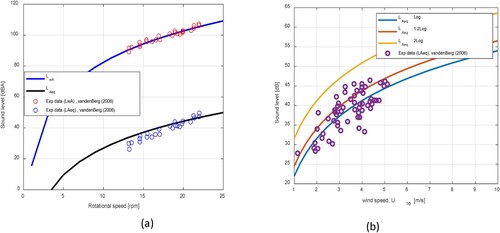
Figure 12. (a) Turbulent boundary layer trailing edge noise computed using BPM, Lowson and Grosveld methods as function of rotor RPM (Bhargava and Samala Citation2019a). (b) Standard error of sound pressure (dBA) between BPM, Lowson and Grosveld methods at U = 8 m/s for increasing rotational speed in RPM.
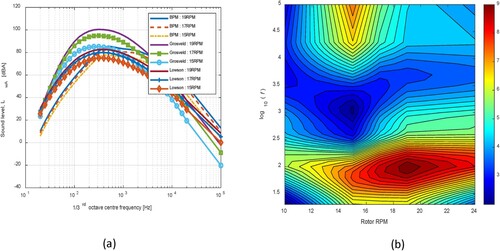