Figures & data
Figure 1. (A) Nanoparticles interact with biological systems at the molecular, cellular, organismal, and ecosystem levels. (B) Computational approaches to understanding the nano–bio interface span orders of magnitude in time and space (A, B reproduced with permission from Murphy et al. Citation2015). (C) The generally accepted principle assesses risk as: Risk = Hazard × Exposure. (D) Workflow of in vitro testing. The nanomaterials are dispersed in a physiological nutrient medium that contains proteins and other macromolecules (coils) and low‐molar‐mass components such as salts (dots). The nanomaterial surface changes by differential adsorption of some of these components, correlated with changes in the state of agglomeration. Only afterwards, the interaction with a multitude of cell species is studied by the (typically optical) readout of a large number of markers and endpoints (C, D reproduced with permission from Landsiedel et al. Citation2015).
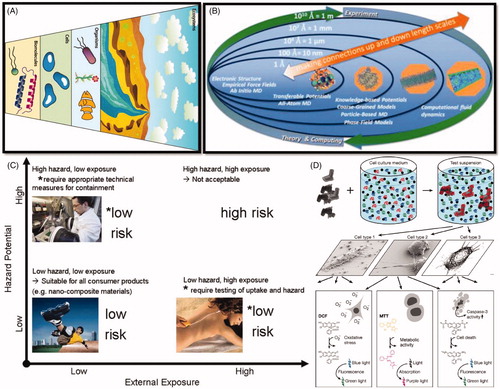
Figure 2. (A) Regulatory bodies in USA and European Union working as watchdog for nanotoxicological assessment. (B) Key scientific considerations to enable understanding of the long-term, medium and short opportunities in nanotoxicology. The boxes on the left hand side detail the tools that are essential towards (i) ensuring that fundamental properties and nanomaterial lifecycle are measured in the prioritization of nanomaterials taken forward into risk testing, and (ii) the efficacious utilization of in vitro, mechanistic methods to predict apical toxic effects. Figure adapted from Burden et al. (Citation2017).
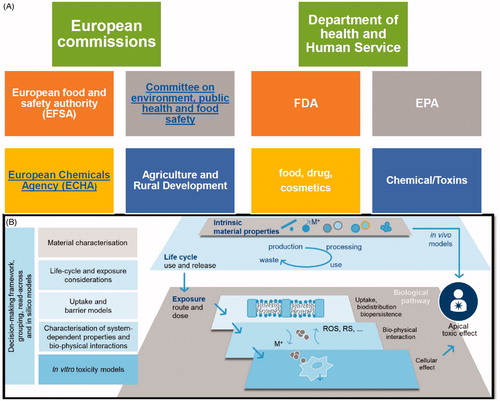
Figure 3. (A) High throughput screening of nanomaterial toxicity in vivo (George et al. Citation2011). (B) Concepts of the combinatorial materials-development workflow. Upper panel with boxes; Initial concept proposed by Hanak (Citation1970). Lower panel demonstrates modern ‘combinatorial materials cycle’ (Potyrailo and Mirsky Citation2008). Reproduced with permission from Hanak (Citation1970) and Potyrailo and Mirsky (Citation2008). (C) Use of compositional and combinatorial ENM libraries including metals, metal oxides, carbon nanotubes, and silica-based nanomaterials, to perform mechanisms-based toxicological screening that links material composition and systematic variation of specific properties to biological outcome (Nel et al. Citation2013). Reproduced with permission from Nel et al. (Citation2013).
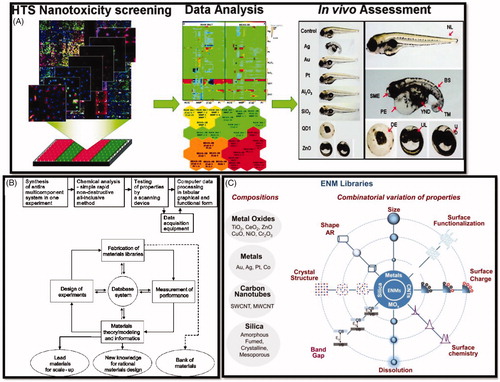
Figure 4. AFM characterization of surface topography and nanoroughness of different TiO2 films. (A–D) Representative height maps in three-dimensional view of ns-TiO2 films with increasing thickness (50, 100, 200, and 300 nm); (E–H) Representative surface profiles exhibiting variations in Rq, Aspec, correlation length, skewness, and kurtosis, as well as in pore width and depth distributions, as discussed in the main text. (I, J) Proposed pathway for new particle formation from parent (nano) particles. (K) Main differences between ionic, nanoparticulate, and bulk silver. Reproduced with permission from Glover et al. (Citation2011), Singh et al. (Citation2011b), Reidy et al. (Citation2013), and Hussain et al. (Citation2015).
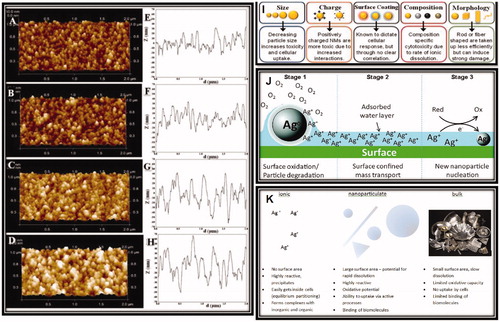
Figure 5. (A, B) Life cycle of a nanocomposite. (A) Relevance for exposure of professionals or consumers, and environmental emission from nanofiller production to end of life/recycling. (B) Specific life cycle map of sports equipment made of MWCNT-polymer composite, detailing release processes that must be considered with the anticipated relative release probability indicated by the thickness of arrows. (C) Schematic illustration of the competing environmental transformation and organismal uptake processes that occur for a nanomaterial in aquatic environments, illustrated using a silver nanoparticle. (D) Characterization of porous nanoparticles for in vitro or post in vivo exposure in blood. Reprinted with permission from Harper et al. (Citation2015), Malysheva et al. (Citation2015), and Valsami-Jones and Lynch (Citation2015).
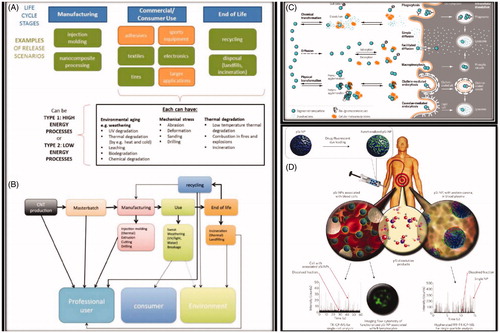
Table 1. Recommendations regarding exposure conditions used in assessing ENM hazard potentials (Holden et al. Citation2016).