Figures & data
Figure 1. Protein expression and subcellular localization of H3T3-(P)during meiotic maturation. Western blot analysis of the protein expression of H3T3-P, H3S10-P and Haspin in mouse oocytes during meiotic division. Each sample was composed with 50 oocytes collected at 0, 2, 7 and 17 h of maturation culture, corresponding to GV, GVBD, MI and MII stages, respectively. GAPDH was included as a protein loading control. Each protein expression was determined at least 3 times.
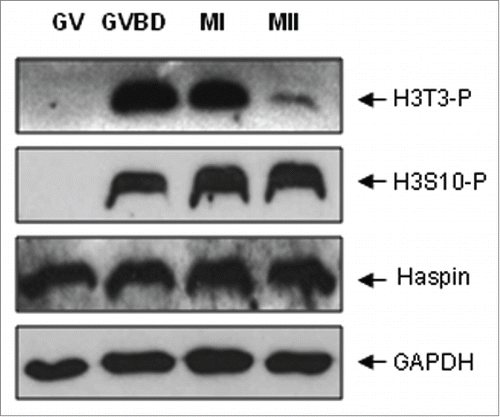
Figure 2. H3T3-(P)subcellular localization in mouse oocytes during meiotic maturation. (A) Oocytes at various stages were immunostained with antibodies against H3T3-P and Haspin. DNA was visualized in blue, H3T3-P in red and Haspin in green. Bar, 20 μM. No special accumulation of H3T3-P or Haspin was detected in oocytes at GV stage. H3T3-P was brighly detected on the condensing chromatin after GVBD, H3T3-P remained localized on chromosome as cell cycle progressed to MII, even during AI-TI transition (s). Haspin began to accumulate on chromosomes upon GVBD, and remained localized on chromosomes as oocytes developed to MI stage (n: arrow), Haspin was also distributed across spindle area (n: arrowhead; v: arrow). During AI-TI transition, Haspin was transferred to the midbody, only weak signal of Haspin was labeled on dividing chromosomes (r). (B) Oocytes chromsome spreads were prepared and immnuo-labeled with antibodies to H3T3-P and human auto serum CREST, which recognizes centromere complex. H3T3-P was visualized in red, CREST in green and DNA in blue. Bar, 10 μM. H3T3-P was localized on chromosome, particularly accumulated along the interstitial axes between chromosome arms at pro-MI and MI stages (a-j). H3T3-P aggregation was dramatically reduced and mainly confined within the local space between sister kinetochores at MII stage (l-n: arrow). In addition, H3T3-P was also weakly labeled on centromeres.
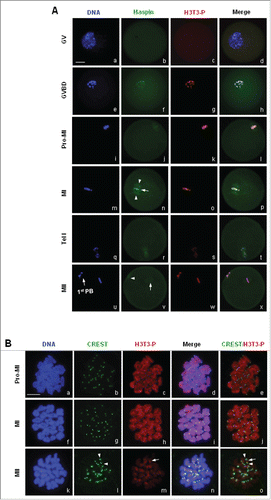
Figure 3. Subcellular localization of H3S10-P in mouse oocytes during meiotic division. (A) Immunofluorescence staining showed dynamic localization of H3S10-P in mouse oocytes during meiosis. Spindle microtubules were visualized by acetylated tubulin in green, DNA in blue and H3S10-P in red. Bar, 20 μM. No signal of H3S10-P was detected in GV oocytes, H3S10-P only began to be detected on the condensing chromatin upon GVBD. H3S10-P accumulation was sustained on chromosome during meiotic progression from GVBD to MII. (B) Oocytes chromsome spreads were prepared and processed for immnuo-labeling with antibodies to H3S10-P and human auto serum CREST. H3S10-P was visualized in red, CREST in green and DNA in blue. Bar, 10 μM. H3S10-P was evenly distributed across the whole chromosome body from pro-MI to MII.
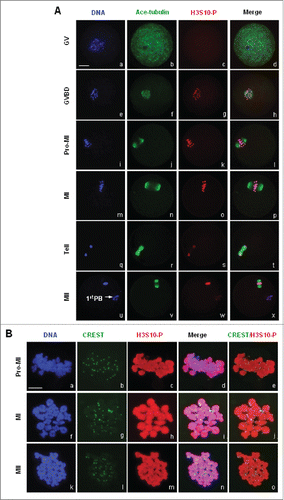
Figure 4. Western blot analysis of reduced H3T3-(P)in oocytes by 5-ITu in a dose and time-dependent manner. (A) Following in vitro maturation for 5 h, oocytes were incubated for additional 1 h in the presence of 0, 0.1, 1 and 5 μM 5-ITu, respectively, prior to western blot analysis. The protein expressions of H3T3-P and H3S10-P were assayed, a control blot of GAPDH shows equal protein loading between the various samples. After 1 h incubation, H3T3-P expression was significantly reduced in 0.1 μM 5-ITu group, and totally disappeared in 1 μM 5-Itu and 5 μM 5-ITu group. The protein level of H3S10-P remained stable in oocytes after treated with different concentrations of 5-ITu. (B) Pro-MI oocytes were incubated with 1uM 5-ITu for 0, 10, 30, and 60 min, respectively, prior to protein gel blot analysis. Both H3T3-P and H3S10-P were determined after drug treatment. GAPDH level was assayed and used as a control blot. H3T3-P protein level was markedly decreased after 10 min incubation in 1 μM 5-ITu, and could not be detected after 30 min treatment. In contrast H3S10-P level sustained consistently at different time points of drug treatment.
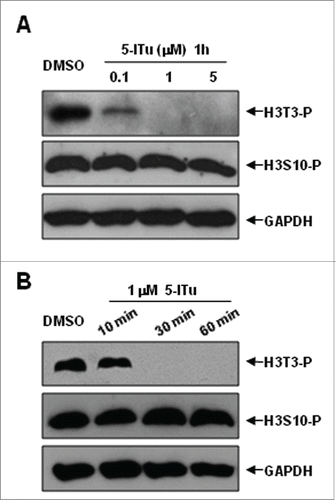
Figure 5. Immunofluorescence analysis of reduced H3T3-P in oocytes by 5-ITu in time- and dose-dependent manner. (A) The subcellular localization of H3T3-P and H3S10-P was analyzed with immunofluorescent staining in pro-MI oocytes incubated for 1 h in 0, 0.1, 1 and 5 μM 5-Itu, respectively. DNA was visualized in blue, H3T3-P or H3S10-P in red and acetylated-tubulin in green. Bar, 20 μM. At the end of 1 h incubation, the fluorescent signal of H3T3-P was significantly reduced in 0.1 μM 5-ITu group, and totally undetectable in oocytes treated with 1 μM 5-Itu and 5 μM 5-ITu. H3S10-P was brightly labeled on chromosomes in oocytes after treated with different concentrations of 5-ITu. (B) The subcellular localization of H3T3-P and H3S10-P was detected with immunofluorescent staining in pro-MI oocytes after treated with 1 uM 5-ITu for 0, 10, 30 and 60 min. DNA was visualized in blue, H3T3-P or H3S10-P in red and acetylated-tubulin in green. Bar, 20 μM. H3T3-P accumulation on chromosomes was dramatically reduced after 10 min incubation, and total undetectable in oocytes treated for 30 min. Bright H3S10-P was consistently detected in oocytes at all the time points of drug incubation.
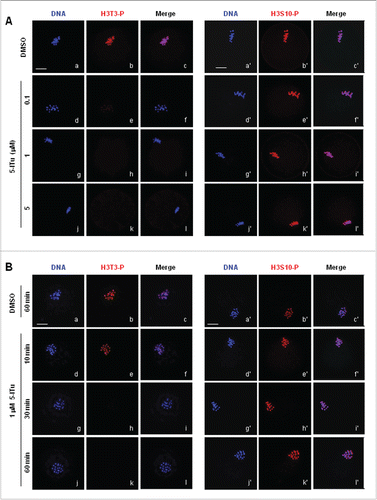
Figure 6. H3 Thr3 phosphorylation involvement in meiotic resumption and cell cycle progression to MII in oocytes. (A) Immunofluorescence analysis demonstrated that non-condensed chromatin after GV oocytes were incubated for 4 h in 5 μM 5-ITu. DNA was visualized in blue, H3T3-P in red and acetylated-tubulin in green. Bar, 20 μM. The nuclear envelope was still intact (e) in 5-ITu-treated oocytes with no signal of H3T3-P expression (g) and microtubule assembly (f), in contrast, GVBD occurred and chromatin was properly condensed into individual chromosomes (a) in control oocytes with bright fluorescent signal of H3T3-P (c) and assembling microtubules (b). (B) Statistical analysis indicated that the meiotic resumption was delayed in GV oocytes when H3T3-P expression was inhibited with 5-ITu. GV oocytes were cultured for 1, 2, and 4 h, respectively, in maturation medium added with 1 and 5 μM 5-Itu, then collected for immunofluorescence staining to judge and score oocytes arrested at GV stage. Data are presented as mean ± SEM of 3 repeated experiments. (C) Statistical analysis showed the meiotic progression to MII was affected when oocytes were incubated in 5-ITU for 15 h after GVBD. GV oocytes were initially cultured for 2 h in normal maturation medium, then incubated for additional 15 h in the same medium supplemented with 0, 0.1, 1 and 5 μM 5-ITu. After incubation, oocytes were collected for immunofluorescence staining to determine the developmetal stages of oocytes. I indicates interphase-specific chromosome configuration, C indicates chromosomes collapsed into “ball” structure. Data are presented as mean ± SEM of 3 repeated experiments.
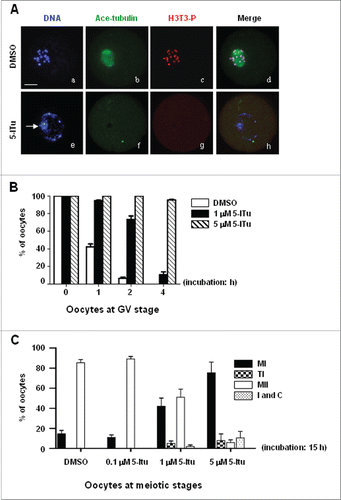
Figure 7. H3 Thr3 phosphorylation was essential for timely MI/MII transition in oocyte meiosis. (A) Statistical analysis demonstrated that inhibition of H3 Thr3 phosphorylation could cause premature start of AI in pro-MI oocytes. GV oocytes were initially cultured for 5 h, then processed for an additional incubation with 1 μM 5-ITu for 1, 2, 3 and 4 h. After that, oocytes were processed for immunofluorescence staining to count the number of oocytes at AI, TI or MII stage. The data represents results of 3 replicates of experiments. (B) Statistical data indicated that H3T3-P inhibition could reverse MI arrest induced by NOCO. GV oocytes were normally cultured in vitro for 7 h, putatively reach MI stage, and then indicated for additional 1, 2, 3 h in the presence of respectively, DMSO, 1 μM 5-ITu, 50 ng/ml NOCO, or 1 μM 5-ITu + 50 ng/ml NOCO, and then processed for immunofluorescence staining to determine specific meiotic stages. Oocytes at AI, TI or MII were assumed to have happened with transition from meiosis I to meiosis II. Graphs report mean ± SEM. All experiments were repeated at least 3 times.
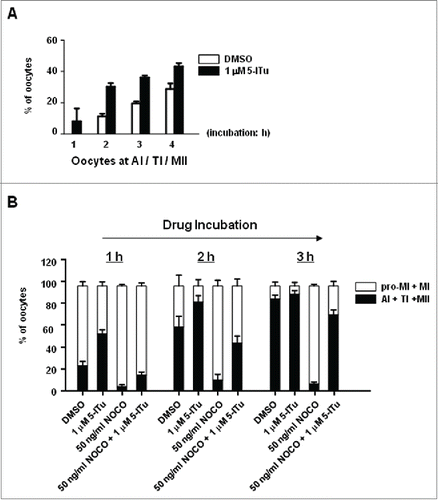
Figure 8. Destroyed recruitment of SAC protein MAD1 to centromeres in 5-ITu-treated oocytes. (A) Immunofluorescence analysis showed disrupted MAD1 accumulation at centromeres in pro-MI oocytes treated with 5-ITu. Oocytes at pro-MI stage were incubated for 1 h in 1 μM 5-ITu, and then processed for chromosome spreading and immunostaining with CREST and MAD1 antibody. DNA was visualized in blue, MAD1 in red and CREST in green. Bar, 20 μM. MAD1 was brightly labeled on centromeres in control oocytes (b-d), but faintly detected in 5-ITu-treated cells (f-h, j-l). (B) Quantization of data indicated the fluorescence intensity of MAD1 was significantly lower in 5-ITu-treated pro-MI oocytes. (C) Immunofluorescence analysis demonstrated that 5-ITu could blocked NOCO-induced MAD1 recruiment to centromeres in MI oocytes. DNA was visualized in blue, MAD1 in red and CREST in green. Bar, 20 μM. MAD1 signal was faintly detected in control MI oocytes (a-d), and undetectable in MI oocytes treated with 5-ITu (e-h). In contrast, MAD1 accumulation on centromeres was markedly increased when MI oocytes were treated with NOCO (i-l), but this increasing recruitment was completely blocked with the combined application of 5-ITu with NOCO (m-p). (D) Statistical analysis indicated that NOCO-induced MAD1 recruiting back to centromeres was blocked with combined administration of 5-ITu. The graph shows the mean ± SEM of the results obtained in 3 independent experiments.
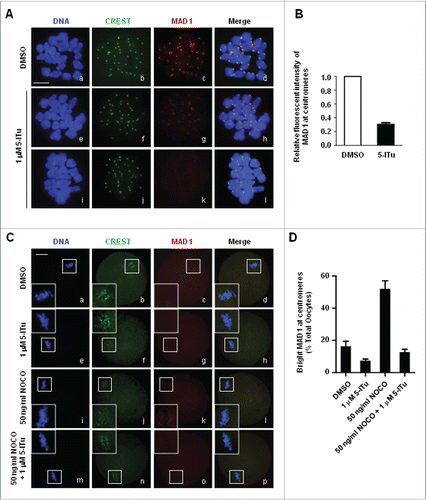