Figures & data
Figure 1. DDT pathways mediated by Rad8, Rhp18, and Rad51 function during the checkpoint response to UV. Asynchronous populations of fission yeast cells were imaged before and after exposure to UV radiation. (A) The average duration of the first cell cycle and the average lengths of cells at the first cleavage are shown for 300 mock-irradiated cells. Error bars denote 95% c.i. (B) Checkpoint responses of mutants in various repair pathways were calculated by subtracting the average length of mock-irradiated cells from the average length of cells exposed to 5 J/m2 of UV (rev3 encodes Polζ). Sample sizes are 300, 600, and 1200 cells for the 1st, 2nd, and 3rd cycles respectively. For mre11Δ and rad51Δ strains, the suppressive effects of spontaneous cell cycle delays were eliminated by restricting analysis to cells < 17 µM at the first cleavage as described in Fig. S2 and in the main text. This procedure reduced the sample size in the mre11Δ and rad51Δ analyses by ∼1/3 and ∼2/3 respectively.
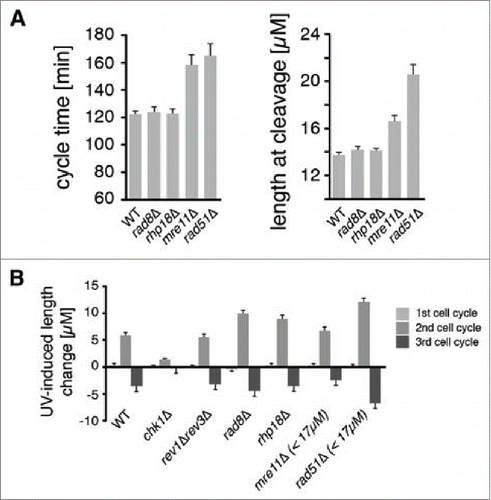
Figure 2. The use of cleavage time to determine cell cycle stage in asynchronous populations. (A) Illustration of the fission yeast cell cycle with sample images from a time-lapse movie of a cell expressing tagged histone H3. C = cleavage/cytokinesis. (B) Determination of the timing of cell cycle landmarks. Confocal microscopy was used to make time-lapse movies of strains expressing GFP fused to α-tubulin (Atb2-GFP) or RPA1 (Rad11-mYFP). Cell cycle landmarks were manually scored as described in Fig. S8. A moving average of the percentage of cells that had passed the indicated landmark was calculated using a 15 min window at increments of 1 min (n = 200 to 300 cells for each experiment). The position of the G2/M checkpoint, determined in panels D and E, is also shown. (C) The percent of cells at the indicated stages were determined from the data in B by calculating the percent of cells that had passed one landmark but had yet to pass the subsequent landmark as described in detail in Fig. S8. (D-F) Cells pass the G2/M checkpoint 1 h before cleavage. Kinetics of the first cleavage event is shown for 300 cells with the indicated genotypes and irradiation conditions (UV or X-rays). (G-H) The checkpoint response to UV occurs prior to mitotic spindle formation. The kinetics of spindle formation and cleavage are shown for 300 cells expressing tagged α-tubulin (Atb2-GFP) imaged after mock irradiation or exposure to 25 J/m2.
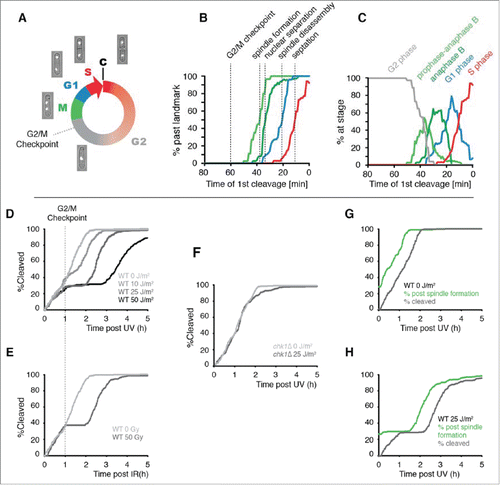
Figure 3. The effects of eliminating DDT pathways on UV-induced checkpoint delays and survival as a function of position in the cell cycle. (A-I) Data from time-lapse movies are plotted as a function of the duration between irradiation and first cleavage, a measure of cell cycle stage at the time of irradiation. Cell cycle stage at the time of irradiation is indicated along the x axis, which was inverted so that cell cycle events appear in temporal order. The beginning of S phase was defined as the point of maximal checkpoint delay in WT cells. A moving average of cell length at the 2nd cleavage, a measure of the checkpoint response, is plotted in the top panels for 600 daughter cells. A moving average of the percentage of cells that continued to divide for 3 generations (viability out of 1,200 potential granddaughter cells) is plotted in the bottom panels. A 15 min window was calculated at increments of 1 min. UV doses were chosen so that the mutant strain viabilities and checkpoint delays were in the dynamic range of the assay (rev3 encodes Polζ; the eso1-cs allele is a Polη catalytic site mutant). For a comparison of each strain at 5 J/m2, see Fig. S6. To deduce the stage of rad51Δ cells at the time of UV and to eliminate the suppressive effects of spontaneous cell cycle delays, we restricted our analysis in F to cells that were < 17 µM at the first cleavage as described in the text. This procedure reduced the sample size by ∼2/3.
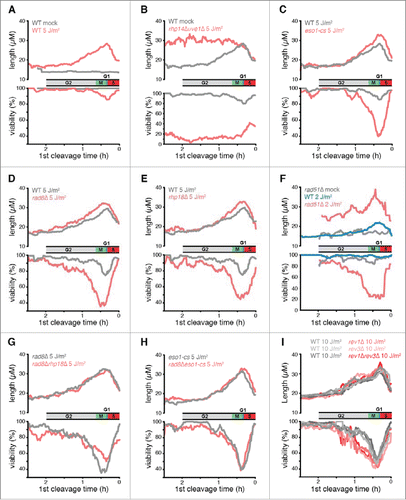
Figure 4. Cells abruptly lose the capacity for HDR of DSBs and begin to exhibit a requirement for mutagenic TLS when they pass the G2/M checkpoint. (A) Rev1 and Polζ are required for survival when damage is incurred between the G2/M checkpoint and mitotic spindle disassembly. The mean and s.d. of the experiments from are plotted. The median times established in are shown for the indicated landmarks. (B) Populations of 300 cells were imaged after exposure to 6.3 Gy of X-rays, a dose chosen to produce 1 DSB/cell on average (0.5 DSB/genome).Citation63 The probability that a genome received one or more DSBs was 0.39 at this level of exposure assuming a Poisson distribution, so 39% loss of viability was expected if each DSB were lethal. Viability of wild-type cells, calculated as in , is plotted as a function of cell cycle stage. Two separate experiments are shown. (C) Wild-type cells exhibit the same X-ray sensitivity as cells that lack HDR when irradiated after the G2/M checkpoint. Loss of viability due to X-ray exposure was calculated by subtracting the fraction of viable cells in an X-irradiated population from that of a mock-irradiated population. Error bars show 95% c.i. The pre-G2/M checkpoint subset represents cells that underwent cleavage 1 hour or more after irradiation, and the post-G2/M checkpoint subset is cells that cleaved less than 1 h after irradiation.
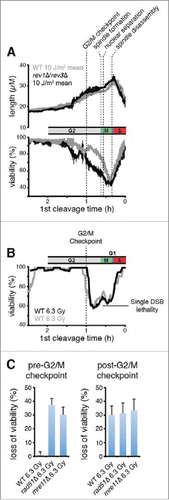
Figure 5. Rad51 is required to prevent Polζ function prior to the G2/M checkpoint. (A) Elimination of Polζ (encoded by rev3) has no effect on the duration of checkpoint delays when UV damage is incurred prior to the G2/M checkpoint in rad51+ cells, but increases delays substantially in rad51Δ cells. Kinetics of the first cleavage are shown for cells of the indicated genotypes exposed to mock irradiation or 25 J/m2 of UV. For strains lacking Rad51, the analysis is restricted to cells with mother cells that were < 17 µM at the cleavage prior to irradiation to reduce the suppressive effects of spontaneous cell cycle delays. (B) UV-induced length increase during the first cycle was calculated for the experiments in A by subtracting the mean length of a mock-irradiated population from the mean length of a population exposed to 25 J/m2 of UV. Error bars denote 95% c.i.
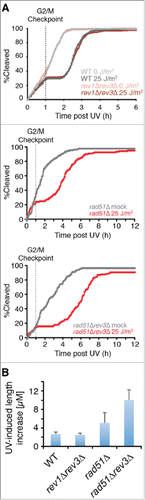
Figure 6. HDR and mutagenic TLS function sequentially and are regulated in concert with checkpoint signaling to maximize the potential for error-free post-replication repair. The DNA damage checkpoint response to UV delays mitosis to allow HDR to complete error-free PRR using redundant information within the sister chromatids. Rad51 recombinase is required to prevent mutagenic TLS by Rev1 and Polζ during the checkpoint delay. A pathway mediated by Rhp18, Rad8, and Polη makes a more limited contribution to PRR at CPDs during the checkpoint delay and may continue to function after the G2/M checkpoint (not shown). After the G2/M checkpoint, cells lose the capacity to complete HDR and rely on Rev1 and Polζ to restore the continuity of the double helix. We suggest that the structures repaired by Rev1 and Polζ are formed most frequently in regions of the genome that remain unreplicated after the G2/M checkpoint. Gaps may form in such regions when replication forks encounter lesions (post-replicative daughter-strand gaps) or when an unreplicated region is unwound during mitosis (mitotic daughter-stand gaps). Rev1 and Polζ may begin to function immediately after cells pass the G2/M checkpoint or, more likely, gain access to DNA when HDR is inactivated at the metaphase-to-anaphase transition.
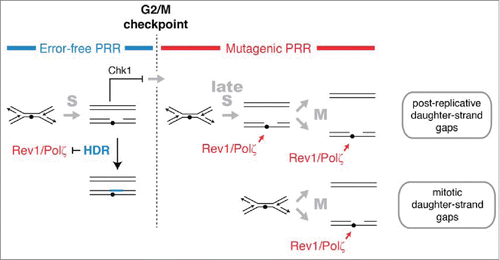