Figures & data
Figure 1. Astrocyte senescence and SASP in neurotoxicity and tauopathy. (a) Summary of our previous findings [Citation7,Citation8] of various triggers of senescence and SASP acquisition in human astrocytes. Using a trans-well co-culture system, we determined that senescent astrocytes exert neurotoxic effects on neurons via upregulation of inflammatory SASP cytokines and loss of neurotrophic factors. Inhibition of SASP by an IL-6 neutralizing antibody prevented astrocyte-mediated neurotoxicity. Conversely, a NGF neutralizing antibody diminished neuroprotective effects of non-senescent astrocytes. (b) Summary of tau propagation in neurodegeneration and our findings on tau-induced astrocyte senescence and SASP (from data shown below in and ). Physiological release of tau and/or pathological lysis of neurons with NFTs could contribute to the accumulation of extracellular tau. Three forms of extracellular tau are used for our experiments: monomers, oligomers (PFF) and their mixture to simulate the formation of larger aggregates. These tau treatments resulted in a phenotypic shift in human astrocytes with an upregulation of IL-6, IL-1β, TNFα, IL-8, and NOS2 (data shown in )
![Figure 1. Astrocyte senescence and SASP in neurotoxicity and tauopathy. (a) Summary of our previous findings [Citation7,Citation8] of various triggers of senescence and SASP acquisition in human astrocytes. Using a trans-well co-culture system, we determined that senescent astrocytes exert neurotoxic effects on neurons via upregulation of inflammatory SASP cytokines and loss of neurotrophic factors. Inhibition of SASP by an IL-6 neutralizing antibody prevented astrocyte-mediated neurotoxicity. Conversely, a NGF neutralizing antibody diminished neuroprotective effects of non-senescent astrocytes. (b) Summary of tau propagation in neurodegeneration and our findings on tau-induced astrocyte senescence and SASP (from data shown below in Figure 2 and Figure 3). Physiological release of tau and/or pathological lysis of neurons with NFTs could contribute to the accumulation of extracellular tau. Three forms of extracellular tau are used for our experiments: monomers, oligomers (PFF) and their mixture to simulate the formation of larger aggregates. These tau treatments resulted in a phenotypic shift in human astrocytes with an upregulation of IL-6, IL-1β, TNFα, IL-8, and NOS2 (data shown in Figure 3)](/cms/asset/62e5424f-1a99-4e50-a400-f8748d5aa2c2/kccy_a_1909260_f0001_c.jpg)
Figure 2. Extracellular tau induces cellular senescence in human astrocytes. (a) Schematic of experimental timeline. Human astrocytes were treated with tau monomers, PFF or combination of both at concentration of 0.1 µg/mL (total tau) for 24 hours and collected immediately or after 3 d. (b) Quantification (percentage) and (c) representative image of SA-β-gal positive cells in untreated control versus astrocytes incubated with tau after 3 d. Significance was determined by unpaired two-tailed Student’s t test (n = 3). (d) Fold change of p21WAF1 mRNA expression after 3 d normalized to GAPDH and presented relative to control. Significance was determined by paired two-tailed Student’s t test (n = 4). (e) Western blots of p16INK4A protein levels. Quantification of p16INK4A levels after 3 d was performed by normalization to GAPDH levels. Statistical significance was determined by paired two-tailed Student’s t test (n = 3). Representative blots are shown on the right. *P ≤ 0.05 and **P ≤ 0.01
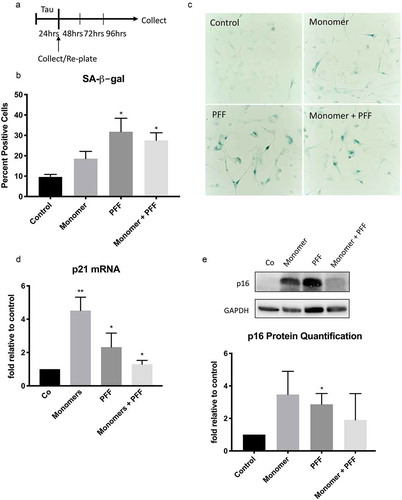
Figure 3. Extracellular tau induces SASP in human astrocytes. Human astrocytes were treated with tau monomers, PFF or combination of both at concentration of 0.1 or 1 µg/mL (total tau) and collected as shown in Figure 1A. All results shown here were from the cells collected after 3 d (Supplementary Figure 1 shows the data at 24 hours). Fold changes of mRNA expression of IL-1β (a), IL-8 (b), NOS2 (c) and TNFα (d) were normalized to GAPDH and presented relative to untreated control. Statistical significance was determined by paired two-tailed student’s t test (n = 3). (e) Representative western blots (top) and quantitative data (bottom) of ASC protein levels in astrocytes treated with 0.1 µg/mL. ASC levels were normalized to GAPDH levels. Statistical significance was determined by paired two-tailed student’s t test (n = 4). (f) Quantification of secreted IL-6 protein in culture media from astrocytes treated with 0.1 µg/mL. Significance was determined by unpaired two-tailed student’s t test (n = 4). (g) Fold changes of mRNA expression of oxidative stress-responsive NRF2 target genes (HMOX1, GCLM, and NQO1) in astrocytes treated with 0.1 µg/mL normalized to GAPDH and presented relative to untreated control. Statistical significance was determined by paired two-tailed student’s t test (n = 4). *P ≤ 0.05 and **P ≤ 0.01
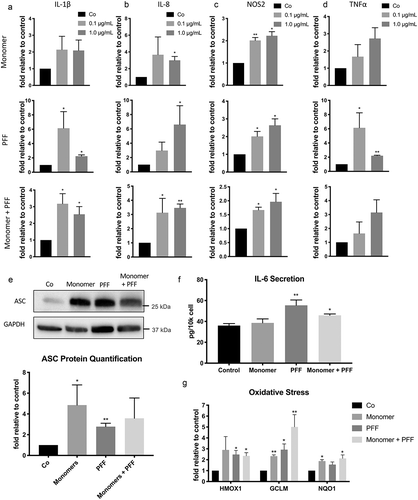
Figure 4. Astrocyte senescence as a central player and therapeutic target in neurodegeneration. Astrocyte senescence is a triggered by a number of stressors leading to neurodegeneration (shown in red). Key initiators of the p53-p21 senescence pathway are DNA damage and oxidative stress. In our previous findings, we showed how senescent astrocytes contribute to neurodegeneration through SASP neurotoxicity and loss of neurotrophic support [Citation7,Citation8] (Figure 1A). SASP promoted inflammation and tau activation of microglia further contribute to the cytotoxic environment and the propagation of tau pathology. Senescent astrocytes also fail to regulate glutamate homeostasis and thereby exacerbate neuronal excitotoxicity. There are several therapeutic avenues targeting astrocyte senescence (listed in green) toward the treatment of neurodegenerative disorders
![Figure 4. Astrocyte senescence as a central player and therapeutic target in neurodegeneration. Astrocyte senescence is a triggered by a number of stressors leading to neurodegeneration (shown in red). Key initiators of the p53-p21 senescence pathway are DNA damage and oxidative stress. In our previous findings, we showed how senescent astrocytes contribute to neurodegeneration through SASP neurotoxicity and loss of neurotrophic support [Citation7,Citation8] (Figure 1A). SASP promoted inflammation and tau activation of microglia further contribute to the cytotoxic environment and the propagation of tau pathology. Senescent astrocytes also fail to regulate glutamate homeostasis and thereby exacerbate neuronal excitotoxicity. There are several therapeutic avenues targeting astrocyte senescence (listed in green) toward the treatment of neurodegenerative disorders](/cms/asset/bc881c8f-e0d1-427a-9f41-f3e798b4ae8a/kccy_a_1909260_f0004_c.jpg)