Figures & data
Figure 1 The classic Venn diagram used to describe the overlapping disease entities included in the definition of COPD (Source: (3), , p. 578).
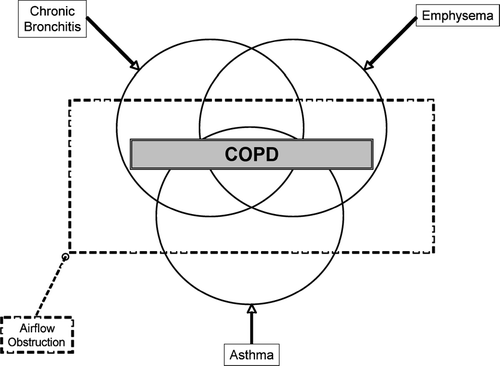
Figure 2 Revised Venn diagram taking into account the expanded heterogeneity of COPD (BMI, body-mass index; ICS, inhaled corticosteroid; BD, bronchodilator).
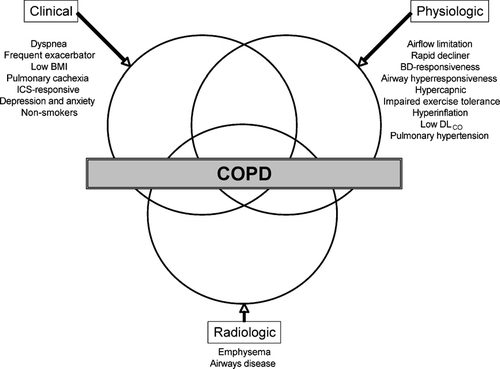
Figure 3 Kaplan–Meier survival curves for the 4 quartiles on the BODE index (Panel A) and the 3 stages of severity of COPD as defined by the ATS (Panel B). Source: (Citation[13]), , p. 1010. In Panel A, quartile 1 is a score of 0 to 2, quartile 2 is a score of 3 to 4, quartile 3 a score of 5 to 6, and quartile 4 a score of 7 to 10. Survival differed significantly among the 4 groups (p < 0.0001). In Panel B, stage I is defined by an FEV1 > 50% predicted, stage II by an FEV1 36–50% predicted, and stage III by an FEV1 ≤ 35% predicted. Survival differed significantly among the 3 groups (p < 0.001).
![Figure 3 Kaplan–Meier survival curves for the 4 quartiles on the BODE index (Panel A) and the 3 stages of severity of COPD as defined by the ATS (Panel B). Source: (Citation[13]), Fig. 1, p. 1010. In Panel A, quartile 1 is a score of 0 to 2, quartile 2 is a score of 3 to 4, quartile 3 a score of 5 to 6, and quartile 4 a score of 7 to 10. Survival differed significantly among the 4 groups (p < 0.0001). In Panel B, stage I is defined by an FEV1 > 50% predicted, stage II by an FEV1 36–50% predicted, and stage III by an FEV1 ≤ 35% predicted. Survival differed significantly among the 3 groups (p < 0.001).](/cms/asset/3274b8e1-afc9-4f00-bf98-02cc7d0451ec/icop_a_262814_uf0003_b.gif)
Figure 4 Body-composition categories are linked to GOLD disease severity. Source: (Citation[29]), , p. 56. Cachexia (N = 117; men, BMI < 21 and FFMI < 16; women, BMI ≥ 21 and FFMI < 5). Semistarvation (N = 23; men BMI < 21 and FFMI ≥ 16; women, BMI < 21 and FFMI ≥ 15). Muscle atrophy (N = 40; men, BMI ≥ 21 and FFMI < 16; women, BMI ≥ 21 and FFMI < 15). No impairment (N = 232; men, BMI ≥ 21 and FFMI ≥ 16; women, BMI ≥ 21 and FFMI ≥ 15). Cachexia was significantly (p = 0.001) more prevalent in GOLD stage IV (N = 207) than in GOLD stage II (N = 71) or III (N = 134). BMI, body mass index (kg/m2); FFMI, fat-free mass index (kg/m2).
![Figure 4 Body-composition categories are linked to GOLD disease severity. Source: (Citation[29]), Fig. 1, p. 56. Cachexia (N = 117; men, BMI < 21 and FFMI < 16; women, BMI ≥ 21 and FFMI < 5). Semistarvation (N = 23; men BMI < 21 and FFMI ≥ 16; women, BMI < 21 and FFMI ≥ 15). Muscle atrophy (N = 40; men, BMI ≥ 21 and FFMI < 16; women, BMI ≥ 21 and FFMI < 15). No impairment (N = 232; men, BMI ≥ 21 and FFMI ≥ 16; women, BMI ≥ 21 and FFMI ≥ 15). Cachexia was significantly (p = 0.001) more prevalent in GOLD stage IV (N = 207) than in GOLD stage II (N = 71) or III (N = 134). BMI, body mass index (kg/m2); FFMI, fat-free mass index (kg/m2).](/cms/asset/f851f366-09e8-44af-aff6-c0109c93ce93/icop_a_262814_uf0004_b.gif)
Figure 5 Prognostic influence of BMI on survival in patients with COPD receiving long-term oxygen therapy. Source: (32), , p. 1463. The initial numbers in each group were 990 (BMI < 20), 1,731 (BMI, 20 to 24), 1,003 (BMI, 25 to 29), and 364 (BMI > 30) [p < 0.001].
![Figure 5 Prognostic influence of BMI on survival in patients with COPD receiving long-term oxygen therapy. Source: (32), Fig. 3, p. 1463. The initial numbers in each group were 990 (BMI < 20), 1,731 (BMI, 20 to 24), 1,003 (BMI, 25 to 29), and 364 (BMI > 30) [p < 0.001].](/cms/asset/60a152a2-7909-4d80-89d7-27100a5f5f27/icop_a_262814_uf0005_b.gif)
Table 1 Systemic effects of COPD
Figure 6 Skeletal muscle apoptosis in COPD. Source: (Citation[51]), , p. 487. Top panel: representative example of a muscle biopsy obtained in a control subject (a) and in a patient with COPD and low BMI (b). Nuclei are stained blue in the former (non-apoptotic), whereas most of them are stained brown (apoptotic) in the latter. Bottom panel: Western blot showing the relative intensities of the two poly(ADP-ribose) polymerase (PARP, a marker of cellular apoptosis) bands (89- and 117-kD fragments) in a representative subject of each of the 4 groups studied.
![Figure 6 Skeletal muscle apoptosis in COPD. Source: (Citation[51]), Fig. 2, p. 487. Top panel: representative example of a muscle biopsy obtained in a control subject (a) and in a patient with COPD and low BMI (b). Nuclei are stained blue in the former (non-apoptotic), whereas most of them are stained brown (apoptotic) in the latter. Bottom panel: Western blot showing the relative intensities of the two poly(ADP-ribose) polymerase (PARP, a marker of cellular apoptosis) bands (89- and 117-kD fragments) in a representative subject of each of the 4 groups studied.](/cms/asset/51415b3a-d074-40aa-a591-81e0b70819ef/icop_a_262814_uf0006_b.gif)
Figure 7 Possible mechanisms of cachexia in COPD. Source: (Citation[37]), , p. 1715. Growth factors such as insulin-like growth factor-I (IGF-I) and MyoD have their anabolic effects by activating myofibrils synthesis. Myofibrillar proteins are marked for degradation by ubiquitin (Ub) and are then processed through the 26 S proteasome. This protein degradation pathway can be activated by muscle inactivity. Proinflammatory cytokines such as TNF (shown here), IL-1, and IL-6, for instance, exert their catabolic action by activating NF-B, which can enter the nucleus after dissociating from its inhibitor (IB). One catabolic action of NF-B is to repress the gene expression of MyoD. Proinflammatory cytokines may also activate the ubiquitin-proteasome pathway. A reasonable hypothesis is that systemic (TNF, growth factors) as well as local factors (inactivity, acidosis) interact in the development of cachexia. Ub = ubiquitin; NF-B = nuclear factor B; IB = NF-B natural inhibitor; MHC = myosin heavy chain; IGF = insulin-like growth factor; IGFR = insulin-like growth factor receptor; TNF = tumor necrosis factor; TNFR I and II = tumor necrosis factor receptor I and II.
![Figure 7 Possible mechanisms of cachexia in COPD. Source: (Citation[37]), Fig. 1, p. 1715. Growth factors such as insulin-like growth factor-I (IGF-I) and MyoD have their anabolic effects by activating myofibrils synthesis. Myofibrillar proteins are marked for degradation by ubiquitin (Ub) and are then processed through the 26 S proteasome. This protein degradation pathway can be activated by muscle inactivity. Proinflammatory cytokines such as TNF (shown here), IL-1, and IL-6, for instance, exert their catabolic action by activating NF-B, which can enter the nucleus after dissociating from its inhibitor (IB). One catabolic action of NF-B is to repress the gene expression of MyoD. Proinflammatory cytokines may also activate the ubiquitin-proteasome pathway. A reasonable hypothesis is that systemic (TNF, growth factors) as well as local factors (inactivity, acidosis) interact in the development of cachexia. Ub = ubiquitin; NF-B = nuclear factor B; IB = NF-B natural inhibitor; MHC = myosin heavy chain; IGF = insulin-like growth factor; IGFR = insulin-like growth factor receptor; TNF = tumor necrosis factor; TNFR I and II = tumor necrosis factor receptor I and II.](/cms/asset/294bf8be-b8de-44d5-af4c-a0f1efdbede3/icop_a_262814_uf0007_b.gif)
Table 2 Prognostic factors in COPD
Table 3 Spirometric classification of COPD severity based on post-bronchodilator FEV1
Table 4 Potential susceptibility genes for rapid decline in lung function
Figure 8 Changes in bronchodilator responder classification and corresponding subgroup mean FEV1 at each visit. Source: (Citation[139]), , p. 662. Numbers in circles refer to the total classified as non-responders at that visit and those in squares are the positive responders on the same occasion. Note that some patients in the ERS criteria group exhibited a “response” after both drugs at the first visit despite being classified as non-responsive to salbutamol alone.
![Figure 8 Changes in bronchodilator responder classification and corresponding subgroup mean FEV1 at each visit. Source: (Citation[139]), Fig. 5, p. 662. Numbers in circles refer to the total classified as non-responders at that visit and those in squares are the positive responders on the same occasion. Note that some patients in the ERS criteria group exhibited a “response” after both drugs at the first visit despite being classified as non-responsive to salbutamol alone.](/cms/asset/374bda75-bfad-4dd3-a27b-5ffd467faee0/icop_a_262814_uf0008_b.gif)
Figure 9 Dynamic hyperinflation in COPD. Source: (Citation[175]), , p. 774. Changes in operational lung volumes are shown as ventilation increases with exercise in patients with COPD and in normal subjects. “Restrictive” constraints on tidal volume (VT, solid area) expansion during exercise are significantly greater in the COPD group from both below (reduced IC) and above (minimal IRV, open area).
![Figure 9 Dynamic hyperinflation in COPD. Source: (Citation[175]), Fig. 3, p. 774. Changes in operational lung volumes are shown as ventilation increases with exercise in patients with COPD and in normal subjects. “Restrictive” constraints on tidal volume (VT, solid area) expansion during exercise are significantly greater in the COPD group from both below (reduced IC) and above (minimal IRV, open area).](/cms/asset/cb012d3f-0cdf-4f88-a8c2-52762125b06f/icop_a_262814_uf0009_b.gif)
Figure 10 Section of a pulmonary arteriole from a patient with PH secondary to COPD. Source: (Citation[192]), , p. 287. All three vessel wall layers are remodeled, with prominent intimal thickening.
![Figure 10 Section of a pulmonary arteriole from a patient with PH secondary to COPD. Source: (Citation[192]), Fig. 1, p. 287. All three vessel wall layers are remodeled, with prominent intimal thickening.](/cms/asset/76a0c874-05c3-47ba-898f-d92f84ac48cf/icop_a_262814_uf0010_b.gif)
Figure 11 Mechanisms of endothelial dysfunction and vascular remodeling in the small pulmonary arteries of patients with COPD. Source: (Citation[185]), , p. 607. Diagram showing various cigarette smoke-related processes that drive vasoconstriction, vasodilation, and cell proliferation in the walls of the small pulmonary arteries. Most of the effects shown are potentially directly mediated by oxidants in the smoke acting on the vessel wall. The vessel is shown in the centre with muscular layer and adventitial layers highlighted. Oxidants in the smoke cause production of the vasoconstrictor endothelin and the vasoproliferative agents endothelin and vascular endothelial growth factor (VEGF). Smoke interferes with nitric oxide mediated vasodilation by decreasing nitric oxide bioactivity. Air trapping as part of chronic airflow obstruction appears to play an early role by inducing vascular compression, and hypoxia causes vasoconstriction, but only when the oxygen tension is extremely low.
![Figure 11 Mechanisms of endothelial dysfunction and vascular remodeling in the small pulmonary arteries of patients with COPD. Source: (Citation[185]), Fig. 2, p. 607. Diagram showing various cigarette smoke-related processes that drive vasoconstriction, vasodilation, and cell proliferation in the walls of the small pulmonary arteries. Most of the effects shown are potentially directly mediated by oxidants in the smoke acting on the vessel wall. The vessel is shown in the centre with muscular layer and adventitial layers highlighted. Oxidants in the smoke cause production of the vasoconstrictor endothelin and the vasoproliferative agents endothelin and vascular endothelial growth factor (VEGF). Smoke interferes with nitric oxide mediated vasodilation by decreasing nitric oxide bioactivity. Air trapping as part of chronic airflow obstruction appears to play an early role by inducing vascular compression, and hypoxia causes vasoconstriction, but only when the oxygen tension is extremely low.](/cms/asset/f746f7a3-fda4-435a-911c-deb3f2eebc5d/icop_a_262814_uf0011_b.gif)
Figure 12 Quantification of airways disease. Source: (Citation[198]), , p. 1103. A representative case showing the process of airway analysis. Using a helical CT image containing the apical bronchus to the upper lobe (A), the algorithm defines the luminal area (B) and wall thickness (C).
![Figure 12 Quantification of airways disease. Source: (Citation[198]), Fig. 1, p. 1103. A representative case showing the process of airway analysis. Using a helical CT image containing the apical bronchus to the upper lobe (A), the algorithm defines the luminal area (B) and wall thickness (C).](/cms/asset/a407b526-0660-48d0-9244-a438fbcfc8a3/icop_a_262814_uf0012_b.gif)
Figure 13 Centrilobular emphysema. (A) a CT through the upper lobes shows multiple poorly defined “black holes”, some of which contain a central vessel, indicating centrilobular emphysema. (B) a similar pattern is seen in the lower lobes of the same patient.
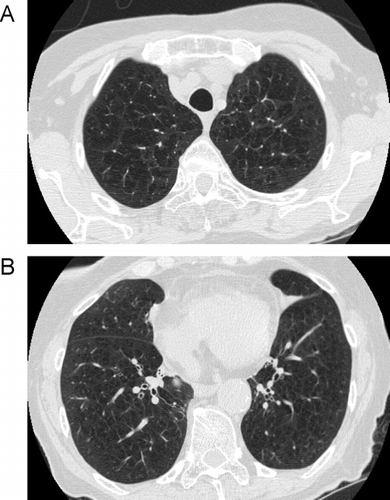
Figure 14 Comparison of centrilobular to panlobular emphysema. (A) the scan through the upper lobes shows some areas of centrilobular emphysema, but there are also some larger areas of decreased attenuation indicating confluent panlobular emphysema. (B) a scan through the lower lobes (same patient as A) shows larger areas of confluent panlobular emphysema.
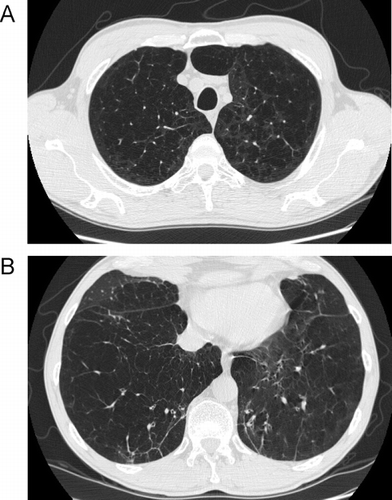
Figure 15 Distal acinar emphysema. Distal acinar emphysema in a tall, thin, 42-year-old cigarette smoker. CT through the lung apices shows predominantly subpleural lucencies indicating distal acinatr (paraseptal) emphysema.
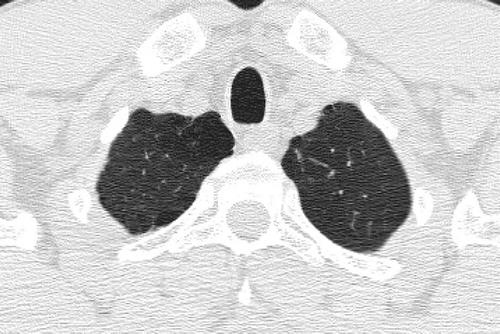
Figure 16 Variable degree of airflow limitation (FEV1) with similar emphysema severity. (A) and (B) are from the same patient, and demonstrate severe panlobular and centrilobular upper lobe emphysema (A), with a predominant centrilobular pattern in the lower lobes (B) but a preserved FEV1. (C) and (D) are from a different patient with a comparable pattern of diffuse upper and lower lobe emphysema. Although there is only slightly worse lower lobe emphysema (D) in the second patient, there is much more severe airflow limitation and loss of DLCO.
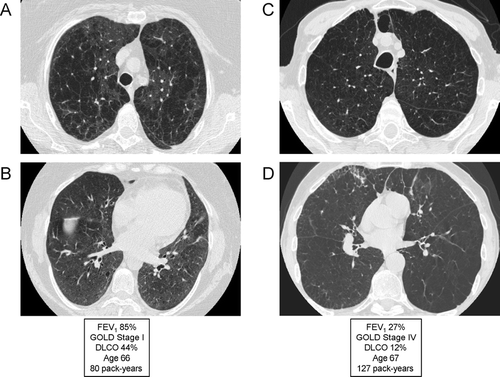
Figure 17 Variable emphysema severity with similar degree of airflow limitation (FEV1). (A) and (B) are from the same patient, and demonstrate mild-to-moderate centrilobular emphysema (CLE) with an upper lobe predominance (A). (C) and (D) are from a different patient, demonstrating moderate-to-severe CLE with an upper lobe predominance (C). Despite the marked visual differences in emphysema severity, the patients are matched for degree of airflow limitation; note that the DLCO correlates better with emphysema severity than the FEV1.
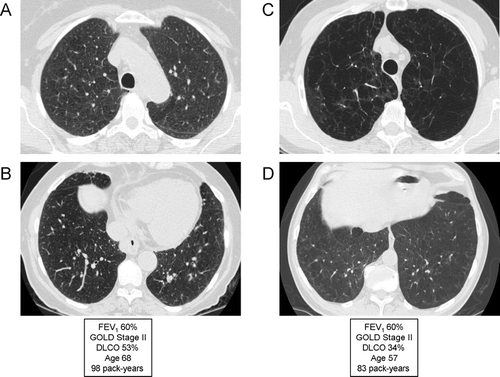
Figure 18 Radiographic appearance of airways disease predominant COPD associated with severe airflow limitation. (A) demonstrates mild upper lobe centrilobular emphysema, while (B) shows very minimal emphysema in the lower lobes but moderate airway wall thickening indicating bronchitis (white arrows) and centrilobular thickening suggesting respiratory bronchiolitis (black arrows).
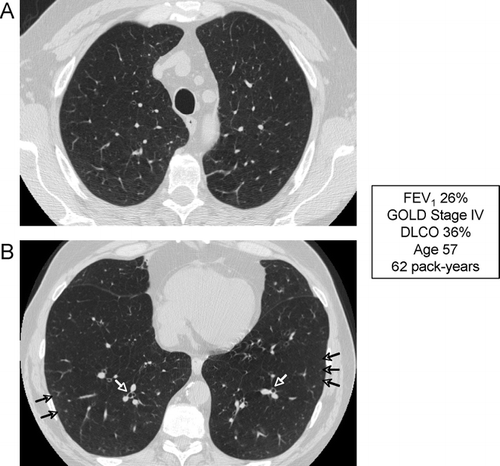
Figure 19 Radiographic appearance of respiratory bronchiolitis-interstitial lung disease (RB-ILD). A 35-year-old heavy smoker with RB-ILD. CT images through through upper (A) and lower (B) lobes show mild centrilobular emphysema, and widespread poorly defined centrilobular nodularity and ground glass abnormality. Focus of decreased attenuation in the right lower lobe is due to air trapping (arrow).
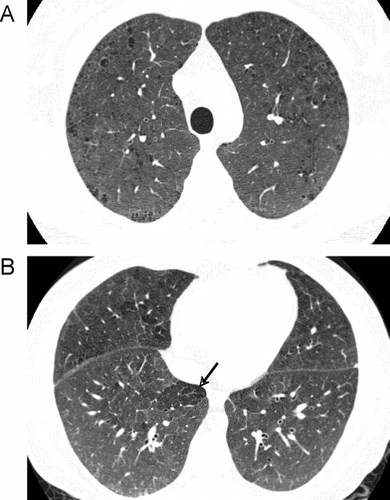