Figures & data
Figure 1. Common methylated nucleotides in tRNA. Sites of methylation are indicated by the red methyl group; abbreviations for the resulting methylated species are shown in brackets in blue. (A) Methylation at C5 of pyrimidines. The bond shaded in red represents a single or double bond in U or C, respectively, and the resulting presence or absence of a proton at N3 is indicated by parentheses. The group at the 4 position (carbonyl in U and amino in C) is indicated by R. (B) Methylation at endocyclic nitrogens of purines and pyrimidines. The red colored bond on the nucleotide base represents a single or double bond, in G/U or A/C, respectively, and the resulting presence or absence of a proton at N3 or N1 is indicated by parentheses. The methylated nucleotides m7G, m1A and m3C are likely to exist as the positively charged species at physiological pH, which has also been experimentally verified for m7G and m1A in the context of an intact tRNA.Citation135 The group at the 6 position of purines (carbonyl in G and amino in A) or the 4 position of pyrimidines (as above) is indicated by R. (C) Methylation at exocyclic nitrogens of purines. Either mono- or di-methylation is observed for both purine nucleotides, as indicated, and the subsequent replacement of one or more protons on the exocyclic amino groups caused by addition of the methyl groups is indicated by parentheses. (D) Methylation at the 2′-oxygen of ribose. A 2′-O-methylated adenosine is shown (Am); each of the other 4 bases, as well as other modified nucleotides can also carry the 2′-O methylation.
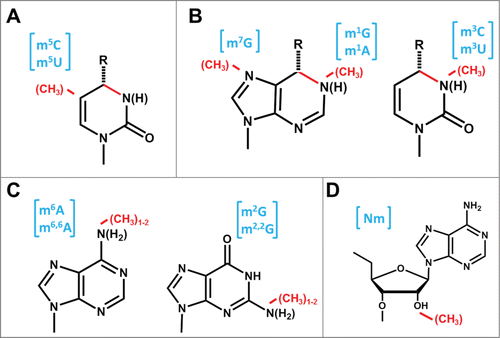
Figure 2. Proposed mechanism for m5U methylation catalyzed by TrmA. Two active site residues (shown in blue) are proposed to act as a nucleophile to attack C5 and create a covalent enzyme-nucleotide intermediate and a general base to remove the proton from C6 following methylation. The methyl donor (SAM) and resulting SAH are shown in red. Although other C5 methyltransferases maintain the cysteine nucleophile, the identity of the general base is not the same for other enzymes; i.e. for Trm4, an Asp is proposed to catalyze this function.
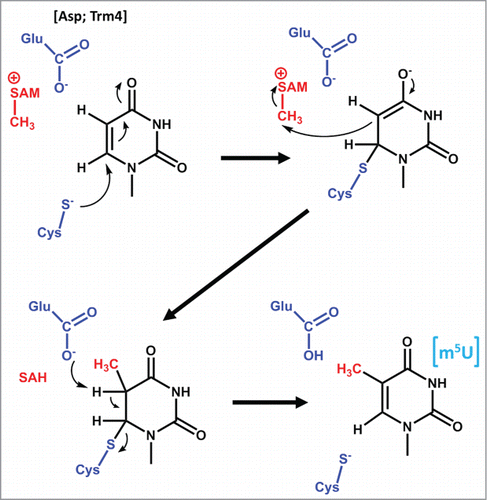
Figure 3. Proposed mechanisms for m1G and m1A formation. (A) Mechanism of m1G37 formation catalyzed by Trm5, with the active site glutamate that serves as the general base indicated in blue. For other m1G methyltransferases, the general base is proposed to be an aspartate, as indicated. (B) Mechanism of m1A58 formation catalyzed by TrmI. The suggested mechanism (based on modeled structures with the target adenosine) involves the action of the aspartate general base (blue) as shown, which may occur during the same elemental step as the attack of N1 on the SAM methyl donor (proposed based on the lower pKa for deprotonation of m1A than for deprotonation of adenosine).
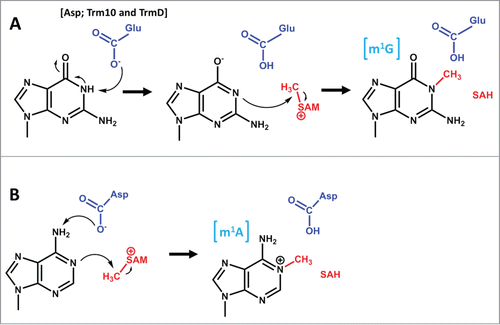
Figure 4. Proposed mechanism for m2G formation by Trm1. The active site aspartate (part of the DPFG motif), which is proposed to serve as a general base to abstract the proton from N2 is shown in blue. An active site tyrosine (not shown) is also proposed to stabilize the activated N2.
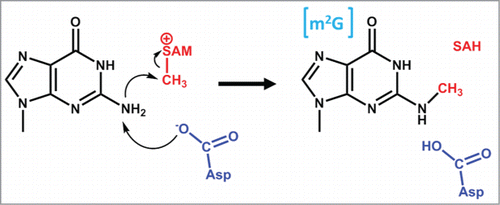
Figure 5. Proposed mechanism for 2′-O methylation of G18 by TrmH. The active site arginine (shown in blue) is proposed both to stabilize the 5′-phosphodiester bond of the target nucleotide, as well as to deprotonate the 2′-OH to promote nucleophilic attack of the 2′-oxygen on the SAM methyl donor.
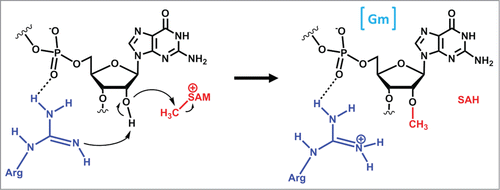
Figure 6. Alternative substrate specificities exhibited by homologous methyltransferases. (A) Dnmt2 catalyzes m5C38 methylation (the C38 target nucleotide is indicated in red), with tRNAAsp thought to be the major substrate for eukaryotic Dnmt2, while tRNAGlu appears to be the predominant substrate for the G. sulfurreducens enzyme. Differences in the size and identity of nucleotides in the variable loop (highlighted in yellow) are thought to play a role in dictating alternative substrate specificity. (B) 2′-O methylation at position 32 is catalyzed by TrmJ. In Bacteria, TrmJ is capable of methylating any nucleotide at position 32 (indicated in red on each tRNA), whereas the archaeal enzyme is restricted to 2′-O methylation of cytosine. Moreover, the different structures required for tRNA recognition by the 2 enzymes (archaeal TrmJ requires only the anticodon stem-loop structure, while bacterial TrmJ requires a full-length tRNA) are indicated by yellow highlighting.
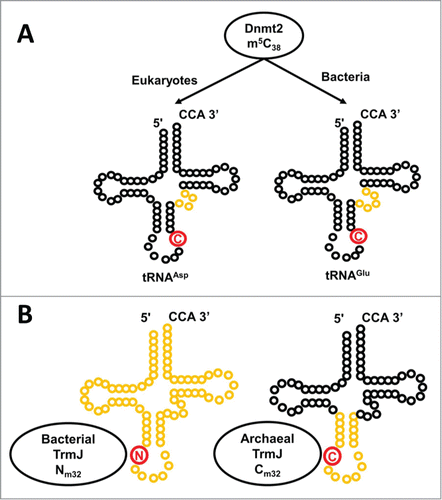
Figure 7. The Trm10 family of methyltransferases exhibit differences in chemistry and substrate recognition. Trm10 enzymes were originally identified based on their ability to catalyze m1G9 methylation (the G9 target nucleotide is shown in red), which only occurs on a subset of tRNAs in S. cerevisiae (left panel). Subsequent investigation revealed that higher eukaryotes encode up to 3 Trm10 paralogs (TRMT10A, B and C, as indicated) and that the methylation activity and substrate specificities of these enzymes are distinct. TRMT10A (left panel) is most similar to S. cerevisiae Trm10 and exhibits similar biochemical properties. TRMT10B (middle panel), like TRMT10A, is a G9 methyltransferase but substrate specificity has not been well characterized, while TRMT10C (right panel) is a strictly mitochondrial methyltransferase with much broader tRNA substrate specificity and the unusual ability to catalyze both G9 and A9 methylation.
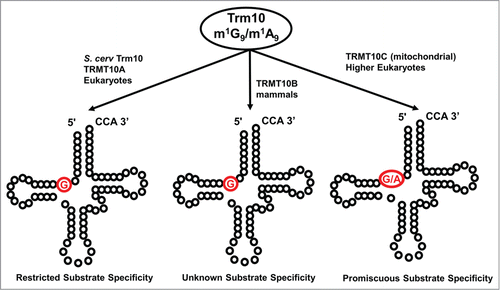
Figure 8. Unusual chemistry associated with tRNA methyltransferase homologs. (A) An archaeal homolog of Trm5 (aTrm5a, also known as Taw22) catalyzes 2 distinct methylation reactions during the formation of the wyosine derivative, mimG. The first reaction, formation of m1G (added methyl group is highlighted in red) is the same reaction catalyzed by other studied Trm5 enzymes, but m1G is subsequently converted to imG-14 (by the action of Taw1) and this modified nucleotide (not shown) is the substrate for the second methylation reaction catalyzed by aTrm5 to form imG2 (the second methyl group added by aTrm5a is shown in red). (B) An unusual SAM-derivative. The metabolite Cx-SAM, with the carboxymethyl group highlighted in red, is attacked by the hydroxyl oxygen at the 5 position of ho5U to generate cmo5U.
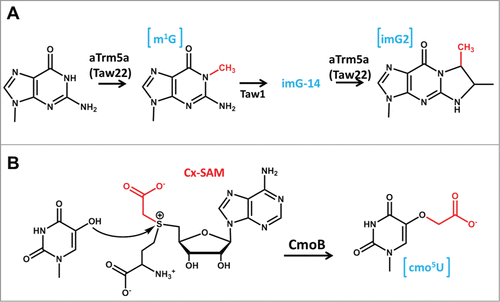
Table 1. Eukaryotic tRNA methyltransferases requiring a partner for efficient catalysis