Figures & data
Figure 1. MIR hairpin stabilization is associated with increased exon skipping. (A) Predicted RNA secondary structure of the MIR exon. The 5′ and 3′ splice sites are shown as blue and red arrows, respectively. Intronic sequences are in lower case, exon positions (upper case) are numbered. A > G mutation is shown by a star. Alignments of FGB intron 1 MIR elements with the MIR consensus is in Figure S1; 4-bp sequence deleted in the antisense MIR copy is shown to the left. Putative branch points are indicated in Figure S1. Target sequences of splice-switching oligoribonucleotides (SSOs) are shown as red lines (5′ and 3′ stem) and a blue curve (loop SSO). (B) Far UV-CD spectrum of the 24-mer hairpin at 25°C showing a strong positive ellipticity at 265 nm. (C) 800 MHz 1H NMR spectrum of the RNA oligo (inset) showing signals for Watson-Crick hydrogen bonded A-U and G-C base pairs between 12–13.5 ppm. (D) FGB minigene reporter. Exons are shown as boxes, introns as lines; their scale is at the top in base pairs. The MIR and T exons are shown as red and blue boxes; the MIR exon-activating mutation is denoted by a star. Dotted lines show canonical (top) and aberrant (bottom) RNA products. MIRs are denoted by blue arrowheads, cloning primers by black arrows and amplification primers by gray arrows. Primer sequences are shown in Table S3. A FGB segment cloned into a hybrid in vitro splicing reporter () is denoted by a black rectangle. (E) Stabilization of the MIR hairpin increases exon skipping. Free energies were computed for the hairpin shown in panel A. A predicted structure of a hairpin destabilising double mutant 13C/14C, which led to full exon inclusion, is shown in the inset. (F) MIR exon inclusion levels for 38 FGB mutations (numbered as in panel A). WT, wildtype minigene; MUT, minigene carrying the disease-causing A > G substitution. All mutations were introduced in the MUT minigene; the same mutations in the WT reporter failed to activate the exon (data not shown). Spliced RNA products (bottom left) are shown as minigene exons throughout; their color corresponds to that in (D). Error bars are SDs of duplicate experiments. Gel panel was merged from separate gels as indicated; cryptic splice sites within or adjacent to the MIR exon were not observed for any construct.
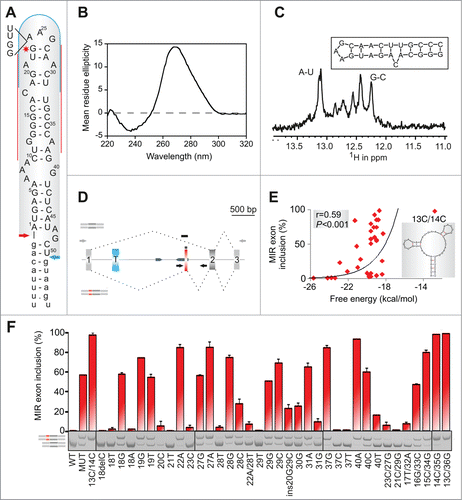
Figure 2. RNA triloops as structural correlates of splicing enhancers and silencers. (A) Mean inclusion levels of 64 terminal triloop mutants of the MIR hairpin exon (top right; mutated residues are in red). Inclusion levels are on a ln scale. Inset shows weighted triloop nucleotide-specific contribution to exon inclusion levels (see Materials and Methods). (B, C) Nucleobase fraction (%) of the indicated categories of splicing enhancers and silencers. Splicing enhancers (B) and silencers (C) were published previously as RESCUE-ESEs,Citation4 exon and intron identity elements (EIEs and IIEs),Citation7 ESEs and ESSs derived by neighborhood inference (trusted NI ESEs and ESSs) Citation77 and QUEPASA ESEs and ESSs.Citation9 (D, E) MIR exon inclusion levels of MIR triloop mutants were best predicted by frequencies of corresponding trinucleotides in QUEPASA hexamers.Citation9 Pearson correlation coefficients and associated P values are shown at the top. Trinucleotide frequencies in QUEPASA enhancers and silencers are shown in Figure S3. Correlation matrix for all enhancer and silencer classes is in Table S1.
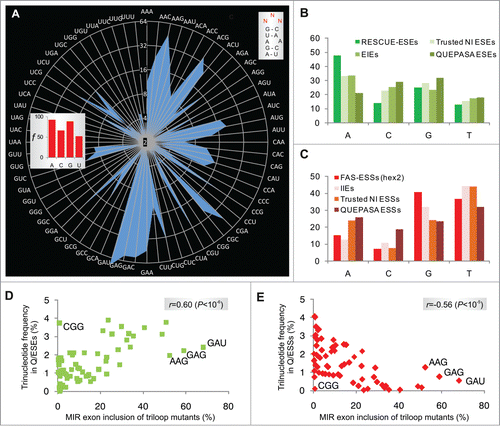
Figure 3. Triloop-dependent promotion and repression of the MIR exon by SSOs. (A, B) Identification of SSOs that target the MIR exon and promote its inclusion in mature transcripts. SSOs are shown at the top, percentage of cryptic splicing (%CS) at the bottom, spliced products schematically to the left and tested triloop sequence to the right. SSO sequences are shown in Table S3 and their hairpin targets in . Final concentrations of each SSO in cell cultures were 40 and 80 nM. GC 5'ss denotes a 2′-O-methyl SSO used as one of the controls; this SSO targets the 5′ splice site of a cryptic exon in the BTK gene and induces exon skipping Citation74 and is also partially complementary to the 5'stem MIR at exon positions 8–10 and 13–17 (). (C) Triloops that promote or repress MIR exon inclusion upon binding of the 5′ stem SSO. A final concentration of the SSO in the culture medium was 50 nM. The panel was merged from 2 separate gels.
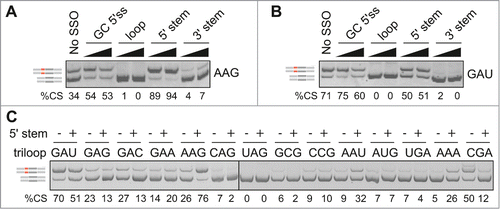
Figure 4. Loop- and stem-dependencies of MIR exon recognition. (A) Hybrid reporter constructs. Exons are shown as boxes (numbered and to a scale shown at the top); introns (not to a scale) are shown as lines. Splicing to a cryptic 5′ splice site in F9 (cr5ss-104) Citation73 is shown by dotted lines. MIR hairpins are in red; r1 and r2 denote their positions in 2 L1CAM constructs. (B) Spliced products of wild-type (WT) reporters and their hybrid counterparts with (MIR MUT) and without (MIR WT) the A > G transition. hd, heteroduplexed PCR products. F9 BP-G constructs had substitution of the branch point adenine for guanine Citation73 to obtain sufficient expression of transcripts skipping exon 3. (C) Exon inclusion levels (%) of terminal triloop mutants in FGB (y-axes) and of corresponding triloop mutants in the indicated hybrid pre-mRNAs (x-axes). Outliers/purine-rich triloops are highlighted. r, Pearson correlation coefficient. (D) Splicing activities of FGB MIR and SMN2 TSL2 triloop mutants. Secondary structure of the native TSL2 hairpin (TSL2/N) Citation21,47 is shown schematically to the left; mutated nucleotides are in red. The 5′ splice site of SMN2 exon 7 is denoted by an arrow. The number of tested triloops is shown at the bottom. (E) Loop-closing base-pairs significantly contribute to the context-dependency of splicing reactions. x-axis shows splicing activities of corresponding triloop mutants in SMN2 TSL2/H. Heptamers shared between MIR () and hybrid (TSL2/H) triloop mutants are denoted by a blue curve; a shared sequence between TSL2/N and MIR is limited to the triloop, as in panel D. (F) A lack of correlation between exon inclusion levels of matching internal and terminal triloop mutants in FGB. Triloops generating high exon inclusion are indicated. (G) MIR exon inclusion levels of internal triloop mutants inversely correlate with the number of hydrogen bonds formed between mutated triloops and the antiparallel strand. The number of hydrogen bonds (x-axis) was calculated from Mfold predictions for each mutant (Table S2). Inset shows that MIR exon inclusion levels (%, y-axis) significantly increased with the number of unpaired bases between the internal loop and the antiparallel strand of the MIR hairpin (P <0.001).
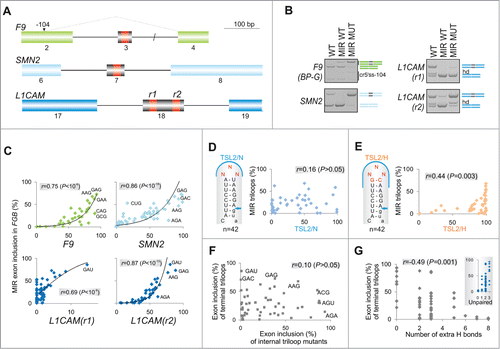
Figure 5. RNA tetraloops that promote and repress MIR exon splicing. (A) MIR exon inclusion levels of 76 constructs with the indicated tetraloops in COS7 cells. RNA products are to the left, merged gels are denoted by vertical lines. Error bars represent SDs from 2 transfections experiments. Stable tetraloops are colored as indicated. A default option of Mfold identified 11 transcripts (marked by symbols at the bottom) with stable alternative RNA secondary structures (shown in panel B); predicted structure of the remaining pre-mRNAs is shown in the inset of panel A.
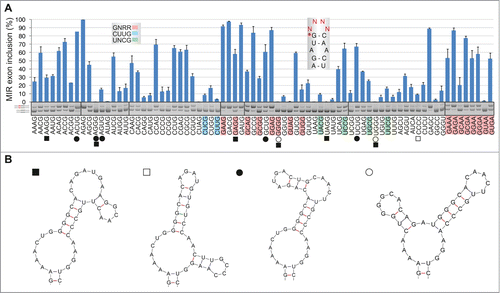
Figure 6. Tra2-induced activation of cryptic exons in FGB intron 1. (A) Activation of the MIR exon is promoted by Tra2. siRNAs targeting the indicated SR or SR-like proteins are at the top, spliced products to the right, FGB reporters are at the bottom. SC1, SC2, scrambled controls. MIR and T exons are denoted throughout as red and blue boxes, respectively. Their position in the pre-mRNA is shown in . SRSF1 and Tra2 immunoblots are shown in panels E and G; depletion levels of endogenous proteins were as described.Citation74,75 (B) T exon activation in cells overexpressing Tra2β. Expression plasmids are at the top; EV, empty vector DNA. (C) Nucleotide sequence of transcripts with T and MIR exons. Sequenced RT-PCR products are color coded as in panel B. (D) Downregulation of SRSF1 promotes MIR exon inclusion. Final concentration and sequence of SRSF1-targeting siRNAs (termed K and N) was as described.Citation75,82 (E) Western blot analysis with anti-SRSF1 antibodies. (1/4), a quarter of the lysate loaded to facilitate estimates of depleted proteins. (F) Distinct role of Tra2α and Tra2β residues R190 and Y/F193 in activation of adjacent exons. The upper panel shows relative positions of mutated residues in the indicated β-sheets of Tra2 RRMs, based on previously published alignments.Citation52,53 The relative abundance of cryptic exons in cells overexpressing wild-type and mutated Tra2 proteins is in the lower panel. Tra2 substitutions are shown at the top. Spliced products (shown to the right) are quantified at the bottom. Concentration of reporter and expression plasmid DNA was 250 and 500 ng/mL, respectively. (G) Immunoblotting of HEK293 cell lysates using antibodies against β-actin and Xpress. Tra2 mutations are at the top. C, no plasmid DNA control. (H) Dose-dependent activation of T- and MIR exons in cells expressing Tra2α R190A and Tra2β R190A. Final DNA concentrations of plasmid expressing Tra2α and Tra2β were 30, 100, 300 and 600 ng/mL, and 6, 20, 60 and 180 ng/mL, respectively.
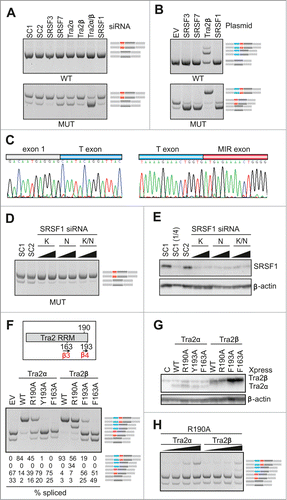
Figure 7. Tra2-induced cryptic exon activation is controlled by the MIR hairpin located >1.5 kb downstream. FGB and hybrid SMN2-MIR constructs were cotransfected with Tra2 expression plasmids and controls (top). The FGB pre-mRNA is shown in and the hybrid SMN2-FGB pre-mRNA in . Spliced products are shown schematically to the right; exons are color -coded as in the pre-mRNAs. Mutated splicing reporters are to the left; mutations were introduced in the terminal triloop (exon position 24–26)/bulge (exon position 18) of the MUT construct, as indicated. WT, wild construct without the 23A > G substitution; EV, empty vector; C, reporter-only controls.
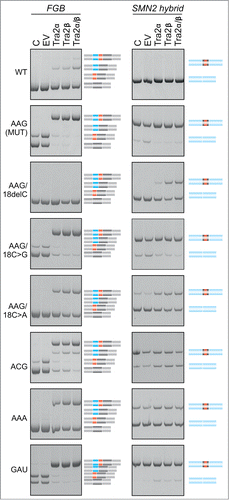
Figure 8. Identification of proteins that bind to PY7-FGB transcripts (A) Schematics of hybrid PY7-FGB reporter construct for in vitro splicing. PY7 Citation54 and MIR () exons are represented by white and black rectangles (to scale at the top), respectively. The A > G mutation is shown by an asterisk. Cloning primers are in Table S3. (B) Splicing of pCR-PY7-FGB hybrid constructs in HEK293 cells. Spliced products are shown to the right. (C) In vitro splicing of WT and MUT PY7-FGB hybrids in HeLa nuclear extracts. 32P-UTP labeled RNAs were incubated in HeLa nuclear extracts for 1 hr. Concentrations of Mg2+ and KCl are at the top, spliced products are to the right. The size of the pre-mRNA/mRNA was 327/201 bp. (D, E) UV-crosslinking of proteins to 32P-UTP-labeled WT and MUT transcripts and their mutated versions. Asterisks correpond to proteins identified by immunoprecipitation in panel F. Panel E shows differential crosslinking to 32P-CTP-labeled bulge mutants at MIR exon position 18. (F) Immunoprecipitation of the crosslinked proteins with the indicated antibodies.
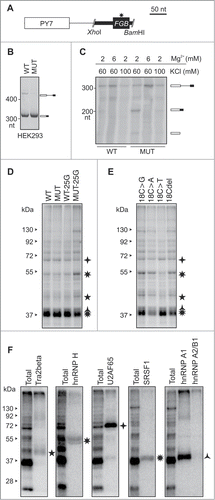