Figures & data
Figure 1 (See previous page). Sequence comparison of the EIF4G3 and EIF4G4 homologues from different trypanosomatids. (A) Clustal W alignment of the EIF4G3 and EIF4G4 homologues from L. major (Lm), T. brucei (Tb) and T. cruzi (Tc), highlighting regions of homology outside the HEAT-1/MIF4G domain. Amino acids identical in more than 60% of the sequences are highlighted in dark gray, while amino acids defined as similar, based on the BLOSUM 62 Matrix, on more than 60% of the sequences, are shown in pale gray. When necessary, spaces were inserted within the various sequences (dots) to allow better alignment. The two candidate eIF4E binding segments mentioned in the text are boxed and numbered 1 and 2. The central MIF4G/HEAT-1 domain, the region which defines the various eIF4G homologues, as well as the putative α-helical rich regions which are likely variants of the MA3/HEAT-2 and W2/HEAT-3 domains are underlined. ▾ indicates the conserved amino acid segments which have been mutated in either L. major or T. brucei EIF4G3 and EIF4G4 in order to investigate their interactions with eIF4E and eIF4A homologues. ⇓ indicates the unique isoleucine/leucine residue which has been identified to be relevant for the interactions between both eIF4G homologues with their eIF4E partners. The * highlights the 2 conserved tryptophan/aromatic residues at the proteins’ C-terminus which resemble equivalent residues within the metazoan HEAT-3/W2 domain. (B) Schematic representation comparing the domain organization of the human eIF4GI with those of L. major EIF4G3 and EIF4G4. For the human protein the sequences implicated in the interactions with PABP, eIF4E, RNA and eIF3 are indicated as well as the 3 defined HEAT domains. For the L. major homologues the positions of the 2 candidate eIF4E binding elements as well as the MIF4G and putative MA3 and W2 domains are also shown.
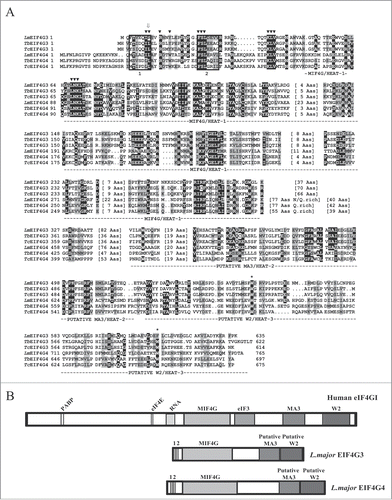
Figure 2. Analysis of the interaction between the L. major EIF4G3 and EIF4G4 and selected partners. (A) Binding interactions between EIF4G3 and EIF4G4 and the EIF4AI homolog. Co-precipitation assays were carried out between a GST-EIF4AI fusion and different [35S]-labeled EIF4G3 and EIF4G4 produced after in vitro transcription and translation reactions. The upper panel shows a representative Coomassie-blue stained gel highlighting the recombinant GST (negative control) or GST fusion protein used in the assays. The panels below show the results from the various assays carried out with the wild-type full-length EIF4G3 and EIF4G4, truncation variants containing only the HEAT-1/MIF4G domain or full-length proteins with the LNK/AAA mutation. “Input” is equivalent to the same amount of labeled proteins (total translation reactions) used in the assays. Numbers on the left indicate the migration of molecular weight markers in kDa. (B) Binding interactions with the 3 Leishmania PABP homologues. Assays were carried out as described in A using recombinant GST-fusioned PABP1, 2 and 3 tested for their ability to bind [35S]-labeled EIF4G3 and EIF4G4. (C) Reverse co-precipitation assays with GST-EIF4G3 and 4 immobilized on the glutathione sepharose beads and incubated with the different [35S]-labeled PABP and eIF4E homologues from L. major.
![Figure 2. Analysis of the interaction between the L. major EIF4G3 and EIF4G4 and selected partners. (A) Binding interactions between EIF4G3 and EIF4G4 and the EIF4AI homolog. Co-precipitation assays were carried out between a GST-EIF4AI fusion and different [35S]-labeled EIF4G3 and EIF4G4 produced after in vitro transcription and translation reactions. The upper panel shows a representative Coomassie-blue stained gel highlighting the recombinant GST (negative control) or GST fusion protein used in the assays. The panels below show the results from the various assays carried out with the wild-type full-length EIF4G3 and EIF4G4, truncation variants containing only the HEAT-1/MIF4G domain or full-length proteins with the LNK/AAA mutation. “Input” is equivalent to the same amount of labeled proteins (total translation reactions) used in the assays. Numbers on the left indicate the migration of molecular weight markers in kDa. (B) Binding interactions with the 3 Leishmania PABP homologues. Assays were carried out as described in A using recombinant GST-fusioned PABP1, 2 and 3 tested for their ability to bind [35S]-labeled EIF4G3 and EIF4G4. (C) Reverse co-precipitation assays with GST-EIF4G3 and 4 immobilized on the glutathione sepharose beads and incubated with the different [35S]-labeled PABP and eIF4E homologues from L. major.](/cms/asset/636bfc5f-c031-41ca-a7a0-df5327ff0d28/krnb_a_1017233_f0002_b.gif)
Figure 3. Fine mapping of the interactions between L. major EIF4G3 and EIF4G4 with their eIF4E partners. (A) Fine mapping of the interactions between L. major EIF4G3 and EIF4E4 and between EIF4G4 and EIF4E3. Co-precipitation assays were carried out as described in using either of the eIF4G homologues fusioned to GST and assayed for their ability to bind [35S]-labeled EIF4E3 and 4. The full-length eIF4G sequences as well as truncations lacking either the N or C-terminal regions of the proteins were used in the assay, as well as site-directed variants where selected residues were replaced by alanines as indicated. The results shown are representative of multiple co-precipitation assays carried out with a minimum of 2 independent sets of GST-tagged proteins. (B) Sequence alignment comparing the eIF4G binding residues (highlighted with a *), previously identified in 3 distinct blocs of the human and yeast eIF4E sequences,Citation23,50 with the equivalent motifs found in different trypanosomatid EIF4E3 and 4 homologues. The alignment was carried out as described in for the eIF4G homologues, but only the segments relevant for the eIF4G interaction are shown. (C) Schematic representation of either known or proposed interactions between different eIF4E/eIF4G homologues. The left scheme summarizes the interactions previously observed, based on the crystal structure,Citation50 between human eIF4E and oligopeptides containing the consensus eIF4E binding motif from human eIF4G and eIF4E-binding proteins (YXXXXLΦ), plus the 3 subsequent residues. The middle and right schemes highlight the likely interactions presumed to occur between the trypanosomatid EIF4E4/EIF4G3 and EIF4E3/EIF4G4 pairs, assuming a conserved mode of binding between the different protein complexes.
![Figure 3. Fine mapping of the interactions between L. major EIF4G3 and EIF4G4 with their eIF4E partners. (A) Fine mapping of the interactions between L. major EIF4G3 and EIF4E4 and between EIF4G4 and EIF4E3. Co-precipitation assays were carried out as described in Fig. 2 using either of the eIF4G homologues fusioned to GST and assayed for their ability to bind [35S]-labeled EIF4E3 and 4. The full-length eIF4G sequences as well as truncations lacking either the N or C-terminal regions of the proteins were used in the assay, as well as site-directed variants where selected residues were replaced by alanines as indicated. The results shown are representative of multiple co-precipitation assays carried out with a minimum of 2 independent sets of GST-tagged proteins. (B) Sequence alignment comparing the eIF4G binding residues (highlighted with a *), previously identified in 3 distinct blocs of the human and yeast eIF4E sequences,Citation23,50 with the equivalent motifs found in different trypanosomatid EIF4E3 and 4 homologues. The alignment was carried out as described in Fig. 1 for the eIF4G homologues, but only the segments relevant for the eIF4G interaction are shown. (C) Schematic representation of either known or proposed interactions between different eIF4E/eIF4G homologues. The left scheme summarizes the interactions previously observed, based on the crystal structure,Citation50 between human eIF4E and oligopeptides containing the consensus eIF4E binding motif from human eIF4G and eIF4E-binding proteins (YXXXXLΦ), plus the 3 subsequent residues. The middle and right schemes highlight the likely interactions presumed to occur between the trypanosomatid EIF4E4/EIF4G3 and EIF4E3/EIF4G4 pairs, assuming a conserved mode of binding between the different protein complexes.](/cms/asset/67c6f27d-1ce6-426d-a390-1025325b34f8/krnb_a_1017233_f0003_b.gif)
Figure 4. Expression analysis and subcellular localization of the T. brucei EIF4G3 and EIF4G4. (A) Quantitation and expression analysis of T. brucei EIF4G3 and EIF4G4 in wild type, exponentially grown, procyclic cells. Recombinant His-tagged EIF4G3 and EIF4G4 were quantitated, diluted to defined concentrations (in fentomoles) and ran on SDS-PAGE gels with whole parasite extract obtained from known number of cells. The gels were then transferred to PVDF membranes and blotted with antibodies directed against T. brucei EIF4G3 or EIF4G4. Densitometric analyses of the results allowed for a rough estimation of the intracellular levels of both proteins, as described.Citation36 (B) Subcellular localization of EIF4G3 and EIF4G4 through indirect immunofluorescence. Procyclic cells were incubated with the same antibodies used in A followed by Alexa Fluor 488-conjugated secondary antibody. DNA was stained with DAPI to identify the nucleus and kinetoplast. (C) The localization of EIF4G3 and EIF4G4 was also confirmed through the expression of eYFP fusion proteins in transfected T. brucei procyclic cells examined under the confocal microscope.
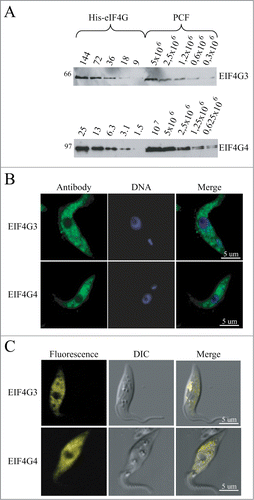
Figure 5. RNA interference of EIF4G3 and EIF4G4. Procyclic T. brucei cells were transfected with the p2T7-177 derived plasmid containing either of the eIF4G genes (EIF4G3 – (A), EIF4G4 – (B). Transfected cells were selected after growth in the presence of phleomycin and RNA interference induced after tetracycline addition. At regular intervals, cellular growth was monitored by counting the number of viable cells of cultures with and without tetracycline and the resulting values used to plot the curves shown (plus tetracycline – gray; minus tetracycline – black). Below each graph are Western-blot analyses of the proteins being targeted, using the affinity-purified antisera specific for each protein. The same blots were then reprobed with affinity purified antibodies against the endogenous control EIF4AI as loading control. All RNAi results shown are representative of multiple experiments performed with distinct transfection events. (C) Effect on cell morphology of the EIF4G4 RNAi preceding cell death. At selected time points after induction of the EIF4G4 RNAi, the cells were visualized at the confocal microscope to monitor for abnormalities in cell morphology. All RNAi results shown in the figure are representative of multiple experiments carried out with a minimum of 2 independently transfected cell lines.
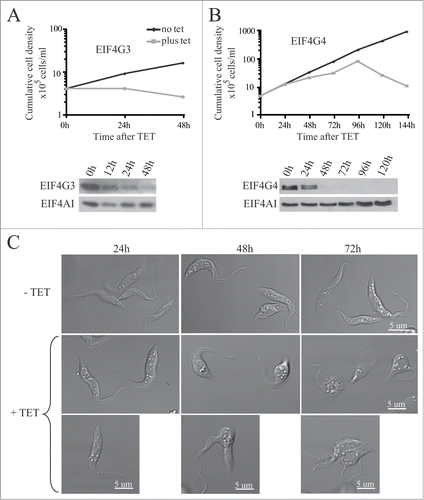
Figure 6. Metabolic labeling of procyclic cells from the RNAi curves of EIF4G3 and EIF4G4. (A) Qualitative analysis of the proteins being synthesized after RNAi. At selected time points after RNAi aliquots of the cells were incubated with [35S]-methionine followed by harvesting and analysis of total protein through SDS–PAGE and autoradiography. (B) Total protein synthesis was also estimated after RNAi for each eIF4G homolog by TCA precipitation and quantitation of the incorporated radioactivity. The results shown are normalized by comparing the incorporation in the RNAi treated cells with the minus tetracycline controls.
![Figure 6. Metabolic labeling of procyclic cells from the RNAi curves of EIF4G3 and EIF4G4. (A) Qualitative analysis of the proteins being synthesized after RNAi. At selected time points after RNAi aliquots of the cells were incubated with [35S]-methionine followed by harvesting and analysis of total protein through SDS–PAGE and autoradiography. (B) Total protein synthesis was also estimated after RNAi for each eIF4G homolog by TCA precipitation and quantitation of the incorporated radioactivity. The results shown are normalized by comparing the incorporation in the RNAi treated cells with the minus tetracycline controls.](/cms/asset/325d1251-d68b-4857-b0f8-7d02851877ab/krnb_a_1017233_f0006_b.gif)
Figure 7. Expression of TY-tagged EIF4G3 and variants in procyclic cells. Growth curves and Western blot analysis of the expression of the TY-EIF4G3 wild-type (A) or the TY-EIF4G3 LNK68-70AAA (B), TY-EIF4G3 FSL24-26AAA (C) and TY-EIF4G3 IL9-10AA (D) variants in transfected cells in the presence or absence of tetracycline (plus tetracycline – gray; minus tetracycline – black). In each case the expression was detected using monoclonal anti-TY and antibodies specific to each of the eIF4G homologues. The blot was simultaneously probed with anti-BiPCitation73 as a loading control. All curves shown are representative of multiple experiments performed with distinct transfection events. The arrows highlight the expression of either the tagged-EIF4G3 proteins (white-filled arrow) or the endogenous EIF4G3 (black-filled arrow). (E) Interaction profile of wild-type TY-EIF4G3, or selected variants, with its EIF4AI and EIF4E4 binding partners. (F) Expression of EIF4G3-eYFP in transfected cells after 48h of tetracycline induction in comparison with the endogenous protein in control cells.
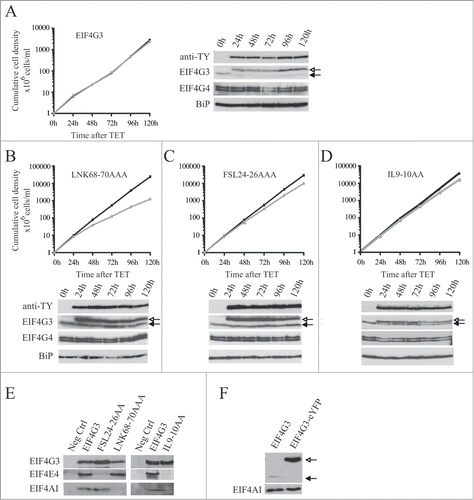
Figure 8. Expression of TY-tagged EIF4G4 and variants in procyclic cells. Growth curves and Western blot analysis of the expression of the TY-EIF4G4 wild-type (A) or the TY-EIF4G4 LNK94-96AAA (B) and TY-EIF4G4 LL27-28AA (C) variants in transfected cells as shown for . (D) Interaction profile of wild-type TY-EIF4G4, or selected variants, with its EIF4AI and EIF4E3 binding partners. (E) Expression of EIF4G4-eYFP in transfected cells after 48 h of tetracycline induction in comparison with the endogenous protein in control cells.
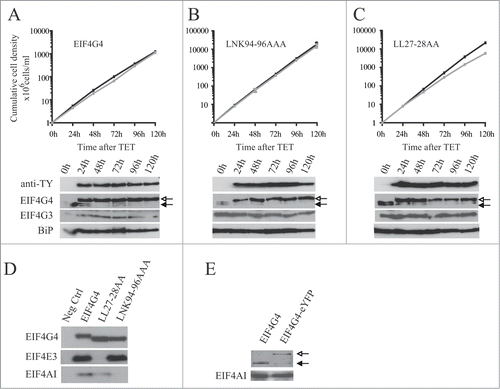