Figures & data
Table 1: Data collection and refinement statistics
Figure 1. (See previous page). Overall Structure of AfCsx3. (A). Sequence alignment of AfCsx3 with various Csx3 proteins from different organisms. The secondary structure elements are shown above the sequence. The α-helices are colored in orange, while the β-strands are colored in green. Conserved residues are shaded in yellow (100% identity), blue (> = 75% identity) and green (> = 50% identity), respectively. Asterisks indicate the critical residues involved in RNA recognition and catalysis. Conserved motif is underlined in red. (B). Stereo view cartoon representation of AfCsx3 showing a ferredoxin-like fold with 6 β-stranded anti-parallel β-sheets located at one side and 2 α-helices resided at the other side. The numbered secondary structures are in the order of appearance in the amino acid sequence. The α-helices are shown in yellow while the β-strands and loop regions are shown in green. (C). AfCsx3 dimer is presented in a cartoon view and the 2 molecules are colored in yellow and magenta, respectively. AfCsx3 dimer aligned by 2-fold non-crystallographic symmetry-related axis. Critical residues which might be involved in dimerization are indicated and colored in cyan. SS-bridge is indicated in green dash line. (D). Superimposition of cartoon and electrostatic potential surface view of AfCsx3 dimer. The dimeric interface harbors a continuously positive-charged groove composed of 4 residues from each molecule. The positive-charged residues are shown as sticks and colored in red.
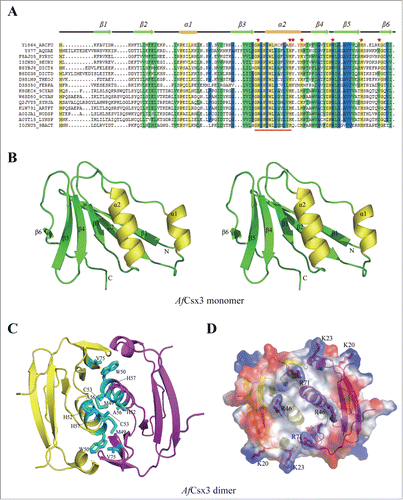
Figure 2. (See previous page). Mn-dependent ribonuclease activity of AfCsx3. (A). Ribonuclease activity displayed by AfCsx3 on repeat ssRNA in the presence of MgCl2, CaCl2 and MnCl2 at 25°C, 35°C and 55°C, respectively. Reaction with no protein added serves as control. The RNA substrates and products are indicated. (B). Ribonuclease activity of AfCsx3 on different nucleic acid substrates. The asterisk indicates the product of cleavable repeat ssRNA. (C).AfCsx3 shows manganese–dependent ribonuclease activity. The manganese-dependent ribonuclease activity is either eliminated by the addition of EDTA or interfered by addition of excessive quantities of Mg2+ or Ca2+ in the reactions. Reaction with no addition of metal ions serves as control. (D). Ribonuclease activity displayed by AfCsx3 on repeat ssRNA in the presence of different divalent cations. (E). Overall cartoon and ribbon view of AfCsx3 in complex with manganese ions. Critical residues involved in the coordination of manganese ions are indicated and colored by element. Manganese ions are indicated as spheres in red mesh contours and colored in wheat. (F). Cartoon view of thecatalytic sites of AfCsx3. Five residues, H57, K58, H60, H80 and E85, are involved in the coordination of 2 manganese ions. Catalytic residues are shown as sticks and colored by element. Manganese ions (Mn A and Mn B) are indicated and colored in wheat. (G). Ribonuclease activity of wild-type and mutant AfCsx3 with mutations on the key residues participating in the coordination of manganese ions. (H). Ribonuclease activity of wild-type and mutant AfCsx3 with mutations on the key residues participating in the dimerization. Reaction with no protein added serves as control.
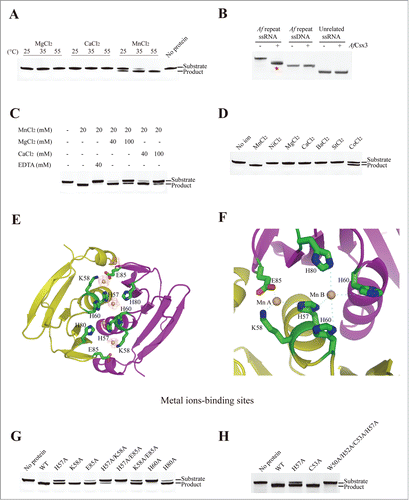
Figure 3. Illustrative model of AfCsx3 bound to RNA. (A). Overall cartoon representation of AfCsx3 in complex with an ssRNA fragment. (B). The cartoon and ribbon (left) and electrostatic potential surface (right) representation of detailed view of RNA binding region. Electron densities for bound ssRNAs are shown in red mesh contours. The map was calculated using Fo-Fc coefficients and phases from the refined structure, but with the RNA residues omitted from the Fc calculation. The map is contoured at 3σ. The ssRNA 5′-N1-N2-N3-N4-3′ is shown in stick model. The key residues involved in AfCsx3-RNA interaction are indicated and colored in red (serving as walls) and cyan (serving as base), respectively.
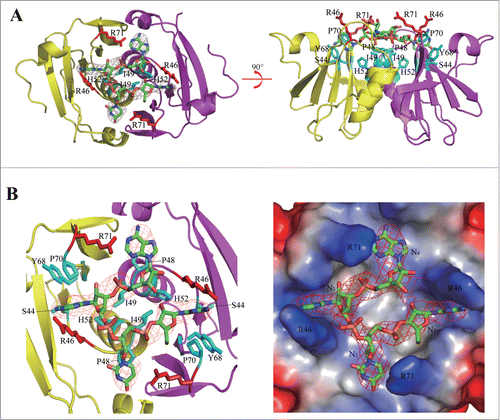
Figure 4. AfCsx3 demonstrates deadenylation exonuclease activity. (A). Subsequent-cleavage assay showing different target sites in repeat ssRNA by AfCsx3 and AfCas6. Solo cleavage by AfCsx3 or AfCas6 and reaction with no protein added serve as controls. The RNA substrates and products cleaved by AfCsx3 and AfCas6 are indicated. (B). Statistical analysis of small RNA library derived from cleaved repeat ssRNA by AfCsx3. Red arrows under and above the sequence indicate the cleavage sites of repeat ssRNA by AfCas6 and AfCsx3, respectively. In consistence with sequencing results, urea-denaturing PAGE showed 4 bands corresponding to the ssRNA substrate and 3 dominant cleavage products (arrows on the gel). The secondary structure of repeat ssRNA was predicted by using the Mfold web server (http://mfold.rna.albany.edu/?q=mfold). (C). Ribonuclease activity of AfCsx3 on different ssRNA substrates derived from CRISPR sequence of Clostridium thermocellum (Ct), Pyrococcus furiosus (Pf) and Thermobifida fusca (Tf), respectively. The asterisks indicate the products of cleavable repeat ssRNAs. (D). Time-course deadenylation assays toward an in vitro transcribed RNA substrate ending with 3′-oligo (A) tail. A7 indicates the intact RNA substrates containing 7 3′ adenylate residues and A0 indicates the fully deadenylated RNA product. The cleavage sites are indicated with arrows above the sequence. (E). Deadenylation assays toward an in vitro transcribed RNA substrate ending with 10 nt 3′-oligo (A) tail. The ladder is shown with a size range from 4˜14 nt. (F). Ribonuclease activity of AfCsx3 on in vitro transcribed ssRNA substrates ending with various types of terminal nucleotides at 3′-oligo (A) tail. (G). Ribonuclease activity of AfCsx3 on ssRNA substrates with various types of terminal 3′-oligo tails.
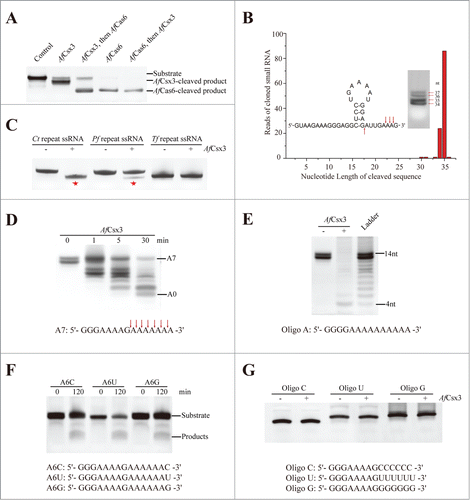
Figure 5. Catalytic mechanisms of AfCsx3. (A). Structural superimposition of AfCsx3 bound to ssRNA fragment (green) and AfCsx3 bound to manganese (cyan). The key residues involved in interaction with ssRNA and in coordination of manganese are indicated and colored in yellow and magenta, respectively. Manganese ions and ssRNA fragment are colored in red. (B). Electrostatic potential surface views of the structural superimposition. The views are the same as those in (a). The surface views show the separated RNA binding site and manganese ions binding site. The positive-charged residues which may contribute to the hypothetical path are indicated. (C). Alternative-syringe model of RNA recognition and cleavage by AfCsx3. The RNA substrate is colored in red. Incoming RNA substrate is recognized by AfCsx3 with the 5′-terminus bound to binding sites (B sites) while the 3′-polyA tail is captured and tethered to active sites (A sites, scissors) for cleavage.
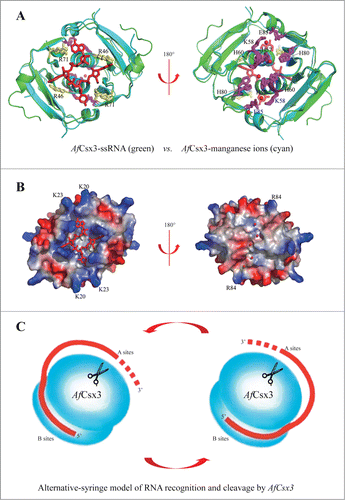