Figures & data
Figure 1. Detection of the small mRNA rreB. (A) Genomic context of rreB and cDNA reads, which originate from a previous differential RNA-seq analysis.Citation19 RNA was isolated from exponentially growing, free-living cells in liquid cultures (F) and from nodules (N). RNA samples were treated (+) or not treated (−) with terminal exonuclease (TEX), which degrades 5′-monophosphorylated (processed) transcripts. The scale of each library is indicated (Reads). Flexed thin black arrow, mapped TSS; dark gray boxes with P and T, mapped putative promoter and terminator, respectively.Citation19 The gene rreB is located between Bjat37 encoding tRNA-Gly and bll5488_sh encoding a 2-component hybrid sensor and regulator. (B) Northern blot hybridization for detection of the sRNA rreB using a probe complementary to the sORF (see ). Total RNA isolated from liquid cultures grown to the exponential growth phase (Exp), the stationary phase (Stat) and from soybean nodules (Nod) was used. On the right side, the positions of the marker RNAs (160 nt, 120 nt and 60 nt corresponding to 6S rRNA, 5S rRNA and a fragment detected by the 6S RNA-specific probe, respectivelyCitation30), are given. The full-length transcript (FL) and putative degradation products (Pr.) were detected. 5S rRNA was used as loading control.
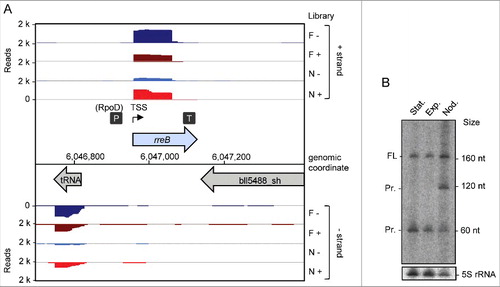
Figure 2. The conserved small RNA rreB contains a sORF and shows extended complementarity to the 3′-end of 16S rRNA. (A) Multiple sequence alignment of rreB mRNA and its homologs in 4 genera of Bradyrhizobiaceae. Asterisks mark invariant positions. The canonical SD sequence 5′-AGGAGG-3′, the GUG start codon and UAA or UAG stop codons are highlighted. The sequence of the 3′-end of 16S rRNA is shown on the top. The 17 nt region of perfect complementarity between rreB and 16S rRNA (17c-region) is framed in blue. Sequences targeted by 3 different probes in Northern blot hybridizations are framed in red (see below). B.j.110, B. japonicum USDA 110; R.p. B18, Rhodopseudomonas palustris BisB18; N.h. 14, Nitrobacter hamburgensis X14; Af. b. 49717, Afipia broomeae ATCC 49717. An extended alignment is shown in Fig. S1. (B) Multiple sequence alignment of the small proteins RreB encoded by the sORFs presented in A). An extended alignment is shown in Fig. S1. (C) Predicted spatial structure of RreB from B. japonicum USDA 110. Aromatic amino acid residues are marked. (D) Predicted RNA secondary structure of the rreB homologs shown in A). The LocARNA color annotation shows the conservation of base pairs - highly reliable prediction is indicated by the red color.Citation36 Less conserved basepairs are indicated by grey letters.
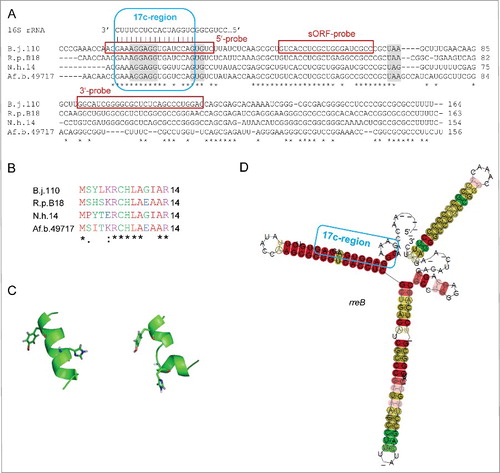
Figure 3. The small mRNA rreB is in the insoluble fraction and is strongly bound to the 30S ribosomal subunit in B. japonicum. (A) Northern blot analysis of total RNA (T) and RNA isolated from the P100 (P) and S100 (S) fraction using probes directed against the 5′-UTR (5′-probe), the sORF (ORF-probe) and the 3′-UTR (3′-probe) of rreB as indicated; the same membrane was re-hybridized. Main bands of approximately 165 nt and 60 nt were detected (marked at the left side). Their lengths were estimated by hybridization of the membrane with probes directed against the length standards 6S RNA (160 nt), 5S rRNA (120 nt) and tRNA-Arg (79 nt) (not shown). (B) Northern blot analysis of tRNA-Arg and 5S rRNA. The membrane shown in A) was re-hybridized. (C) Separation of the P100 fraction through a 5% to 40% sucrose density gradient. The absorption of the gradient fractions at 260 nm is shown. Fractions which were further analyzed are marked with arrows. (D) Analysis of RNA isolated from fractions 3, 10 and 14 of the sucrose density gradient by electrophoresis. RNA was separated in urea-containing 10% polyacrylamide gel and stained with ethidium bromide (shown is a negative image). Distinct bands corresponding to the large 23S rRNA fragment (23S rRNA), 16S rRNA, the 5.8S rRNA-like short 5′-fragment of 23S rRNA, 5S rRNA and tRNAs are indicated. Fragmentation of 23S rRNA in Bradyrhizobium japinicum was described previously.Citation40,41 (E) Northern blot hybridization of RNA from the indicated sucrose density gradient fractions with probes directed against the sORF of rreB, tRNA-Arg and 5S rRNA.
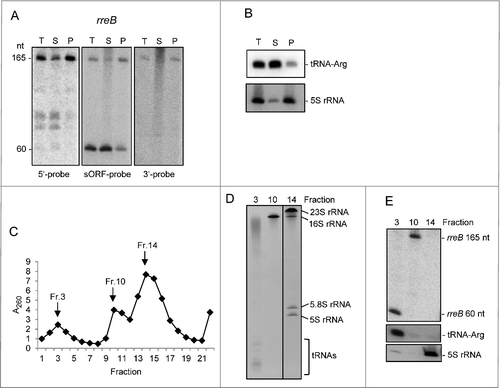
Figure 4. The 5′-nucleotides of the 17c-region are crucial for the predominant localization of rreB in the P100 fraction. (A) Schematic representation of the used constructs. The 5′-UTRs and GTG start codons of the rreB derivatives overexpressed from plasmids pJH-17c-rreB, pJH-14c-rreB, and pJH-8c-rreB are shown. The region of complementarity to 16S rRNA is underlined in blue. The canonical SD and the GTG start codon are highlighted. Prrn, rRNA (rrn) promoter; Trrn, rrn terminator. (B) Northern blot hybridization of total RNA of B. japonicum strains overproducing the indicated rreB derivatives. EVC, empty vector control. (C) Northern blot hybridization of RNA isolated from the P100 (P) and S100 (S) fractions of strains overproducing the indicated rreB derivatives. (B) and (C) shown results from representative experiments. In each case, 3 independent biological experiments with similar results were performed. Probes directed against rreB and the 5S rRNA control were used.
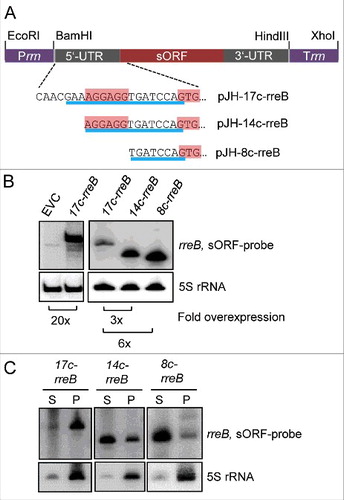
Figure 5. Analysis of the influence of truncated rreB 5′-UTRs on EGFP translation. (A) Schematic representation of plasmid pJH-F2 and of its derivatives pJH-14c-egfp, pJH-11c-egfp, and pJH-8c-egfp, which contain rreB-egfp translational fusions. The standard SD and the ATG start codon of pJH-F2 were replaced by the indicated rreB sequences. n.c., cloning of a construct with the full rreB 5′-UTR without the rreB sORF failed. Prrn and Trrn, see . (B) Western blot analysis with GFP-specific antibodies of strains containing the indicated constructs (see A). EVC, strain containing plasmid pJH-O1. The bottom panel shows a Coomassie Blue stained SDS-polyacrylamide gel after electrophoresis visualizing the loaded protein amounts. Migration of marker proteins (M) in the gel is indicated at the left side in kDa.
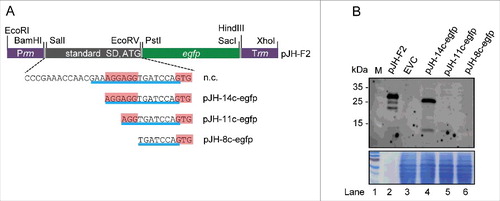
Figure 6. The mRNA rreB is weakly translated in B. japonicum. (A) Schematic representation of the used constructs cloned between a Paph promoter and a sequence for integration of the plasmids into the chromosome (int).Citation29 The rreB-egfp fusion is preceded either by a standard SD and an ATG start codon (pRJ-sSD-rreB-egfp) or by the full-length 5′-UTR of rreB with the 17c-region and the GTG start codon (pRJ-17c-rreB-egfp). Further modifications of the 5′-UTR of rreB resulting in pRJ-14c-rreB-egfp and pRJ-13c-ATG-rreB-egfp are indicated on the bottom. (B) and (C) Western blot analysis with GFP-specific antibodies of strains containing the indicated constructs (see A). EVC, plasmid pJPaph-MCS was integrated into the chromosome. Schematic representation of of pJH-14c-GTG-egfp is given in . The bottom panel shows a Coomassie Blue stained SDS-polyacrylamide gel after electrophoresis visualizing the loaded protein amounts. Migration of marker proteins (M) is indicated (in kDa). Arrows indicate the position of the RreB-EGFP band; arrows with asterisks indicate putative degradation products.
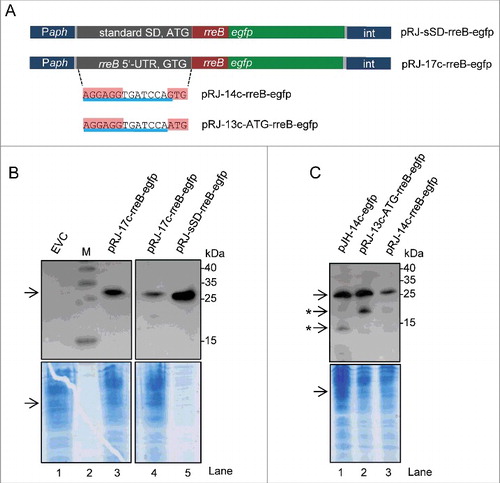
Figure 7. The rreB start codon is important for the turnover of the small mRNA. Northern blots hybridized with probes directed against the rreB sORF and the control 5S rRNA are shown. (A) The 16c-rreB(GUG/UAG) transcript is localized predominantly in the P100 fraction. (B) and (C) RNA was isolated from cells harvested at the indicated time (min) after stop of transcription by rifampin addition to cultures at an OD600 of 0.5. Stabilities of the overproduced rreB derivatives 16c-rreB(GUG/UAG) and 17c-rreB were calculated from 4 biological experiments and are given below the panels.
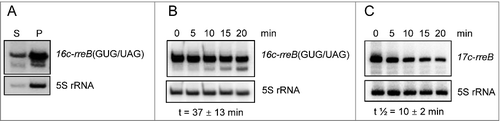
Figure 8. Putative rreB homologs in Rhodobacterales. (A) Multiple sequence alignment of sequences corresponding to the putative small mRNAs containing homologous sORFs of 18 codons (named rreR) and preceded by a region with extended complementarity to the 3′-end of 16 sRNA (Extended SD region). Canonical SD, GTG start codon and stop codons are highlighted in gray. For other descriptions see . (B) Multiple sequence alignment of the small proteins RreR encoded by the sORFs shown in A). O.ar.238, Octadecabacter arcticus 238; O.an.307, Octadecabacter antarcticus 307; J.sp.1, Jannaschia sp CCS 1; Ros.e.19469, Roseibacterium elongatum DSM 19469; Ro.d.114, Roseobacter denitrificans OCh 114; Ro.l.149, Roseobacter litoralis Och 149; D.s.12, Dinoroseobacter shibae DFL 12; P.t.23, Planktomarina temperata RCA 23; Ph.g.26640, Phaeobacter gallaeciensis DSM 26640; Ph.i.17395, Phaeobacter inhibens DSM 17395; Pa.a.7686, Paracoccus aminophilus JCM 7686; R.c.1003, Rhodobacter capsulatus SB 1003; Pa.d 1222, Paracoccus denitrificans PD 1222; Rh.s.2351, Rhodovulum sulfidophilum DSM 2351; C.i.P73, Celeribacter indicus strain P73; R.s.2.4.1., Rhodobacter spaheroides ATCC 2.4.1. (C) Predicted spatial structure of RreR from D. shibae DFL 12.
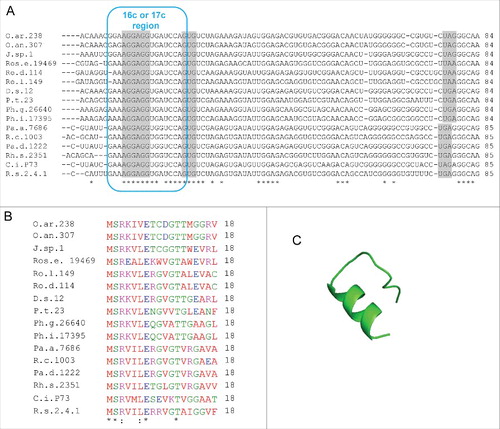