Figures & data
Figure 1. PAX3-FOXO1 during human MSC muscle differentiation. (a) shRNA targeting PAX3-FOXO1 (shPF) specifically silenced the fusion RNA (PF), but not the parental genes. The target sequence has been reported before [Citation8]. The levels of the transcripts were measured by quantitative RT-PCR, and normalized to that of an internal control, GAPDH. (b) MSC cells stably infected with the virus expressing shPF resulted in reduced expression of MYOD, and no expression of MYOG and MYH1. (C-E) Overexpressing PAX3-FOXO1 promotes human MSC cells proliferation and survival. Cell proliferation and viability was measured by MTT. Compared with empty vector transfected cells (hMSCs/CT), human MSC cells stably expressing PAX3-FOXO1 (hMSCs/PF) grew faster in growth media (GM) (c), continued to proliferate in differentiation media (DM) (d), and survived more in serum-free media (SF) (e). *, p < 0.05; **, p < 0.01; ***, p < 0.001.
![Figure 1. PAX3-FOXO1 during human MSC muscle differentiation. (a) shRNA targeting PAX3-FOXO1 (shPF) specifically silenced the fusion RNA (PF), but not the parental genes. The target sequence has been reported before [Citation8]. The levels of the transcripts were measured by quantitative RT-PCR, and normalized to that of an internal control, GAPDH. (b) MSC cells stably infected with the virus expressing shPF resulted in reduced expression of MYOD, and no expression of MYOG and MYH1. (C-E) Overexpressing PAX3-FOXO1 promotes human MSC cells proliferation and survival. Cell proliferation and viability was measured by MTT. Compared with empty vector transfected cells (hMSCs/CT), human MSC cells stably expressing PAX3-FOXO1 (hMSCs/PF) grew faster in growth media (GM) (c), continued to proliferate in differentiation media (DM) (d), and survived more in serum-free media (SF) (e). *, p < 0.05; **, p < 0.01; ***, p < 0.001.](/cms/asset/800ca72a-1567-499b-9c4d-20cd9c1938d3/krnb_a_1564464_f0001_oc.jpg)
Figure 2. Pax3-Foxo1 fusion RNA is not detected in mouse muscle differentiation/regeneration systems. (a) Mouse myoblast cell line C2C12 were induced to differentiate along a skeletal muscle lineage. RNA was extracted from samples harvested from 4 to 96 hours. (b) Bone marrow-derived mesenchymal stem cells, D1-MSCs, were induced to differentiate along a skeletal muscle lineage. RNA was extracted from samples harvested every other day from day 2 to day 22. (c) Mouse embryonic stem cell line, E14, was induced to differentiate along a skeletal muscle lineage. RNA was extracted from samples harvested from day 1 to day 8. (d) Pax3–Foxo1 RNA is absent in mouse fetal muscle samples. RNAs were extracted from fetal (muscle) samples harvested every other day from embryonic day 8 to day 20. (e) A time course of muscle regeneration was induced in the tibialis anterior (TA) muscles by cardiotoxin (CTX) injection. RNA was extracted from the muscle samples collected from day 1 to day 7. In all above, RNAs from the rhabdomyosarcoma cell line RH30 or mouse myoblast cell line C2C12 were used as controls. Pax3–Foxo1, MyoD, MyoG, Myh1, and Gapdh RNAs were assessed by RT-PCR. Lower panels are immuostaining of EDU on CTX or PBS treated muscle slices.
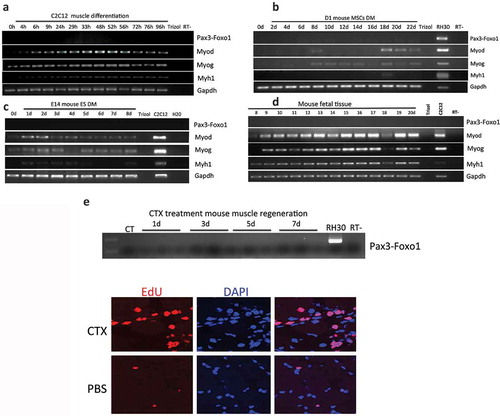
Figure 3. Pax3-Foxo1 fusion RNA is not detected in sheep, pig, or horse muscle differentiation systems. (a-c) Absence of Pax3–Foxo1 RNA during muscle differentiation in sheep bone marrow MSCs (a), pig bone marrow MSCs (b), and horse ASC cells (c). These cells were induced to differentiate along a skeletal muscle lineage. RNA was extracted from samples harvested every other day from day 2 to day 20. Pax3–Foxo1, MyoD, MyoG, Myh1, and Gapdh RNAs were assessed by RT-PCR.
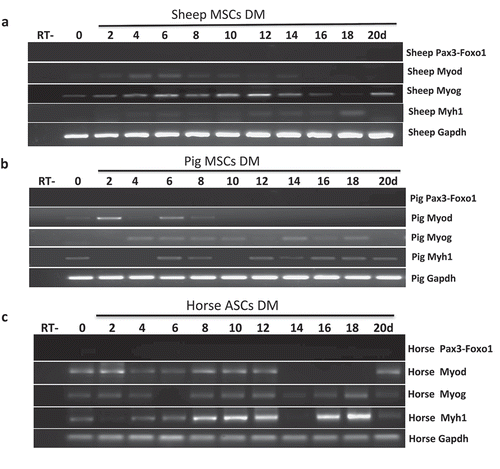
Figure 4. Comparison of wild type Pax3 RNA level. (a) Comparison of wild-type Pax3 RNA levels in MSCs and ASCs of different species. RNA was extracted from human MSCs, mouse MSCs, mouse ASCs, horse ASCs, pig MSCs, and sheep MSCs. Pax3 was measured by qRT-PCR, and its level was normalized against the internal control, Gapdh. Pax3 and Gapdh primers annealing to the conserved sequences among these species were used. (b) Comparison of Pax3 RNA expression during muscle differentiation in mouse bone marrow-derived MSCs and mouse ASCs, with human MSCs. (c) Comparison of Pax3 RNA expression during muscle differentiation in sheep MSCs, pig MSCs, and horse ASCs, with human MSCs. RNA was extracted from samples harvested every other day from day 2 to day 22. *, p < 0.05; **, p < 0.01; ***, p < 0.001.
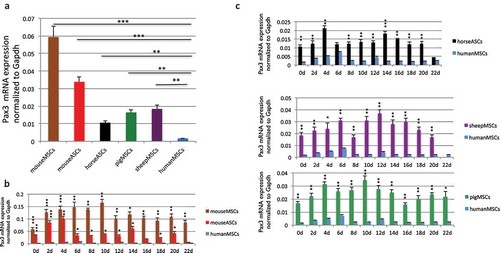
Figure 5. PAX3 is subject to human–specific regulation of microRNA(s) during muscle differentiation. (a) Flowchart for narrowing down the microRNAs that specifically regulate human PAX3, but not mouse Pax3. (b) 16 candidates predicted by TargetScan were examined by luciferase reporter assays. Human PAX3, or mouse Pax3 3ʹUTR was cloned into the miR-Sens plasmid. These plasmids were transfected into HEK293T cells, and subsequently, microRNA mimics were transfected. Six microRNAs that showed suppression on the human construct, but not on the mouse construct are marked with stars. (c) Three microRNAs were further eliminated due to the absence of expression during the human MSC muscle differentiation. qRT-PCR was performed to quantify the level of microRNAs throughout the differentiation process. (d) Mutagenesis at the microRNAs target site. Both miR-495 and miR-382 downregulated the mouse Pax3 reporter when the sequence was changed into the seed sequence of humans. Both also caused upregulation of the human PAX3 reporter when the sequence was mutated to that of mice. miR-186 did not cause downregulation of mutated mouse reporter, even though it did not suppress the mutated human reporter. *, p < 0.05; **, p < 0.01; ***, p < 0.001.
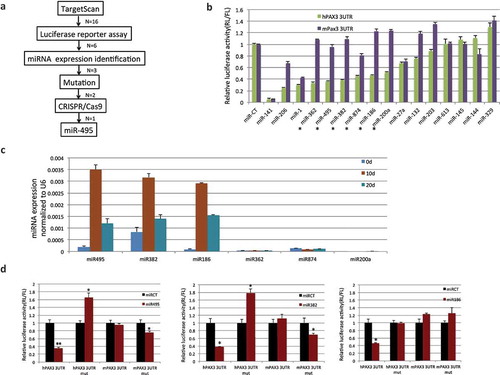
Figure 6. CRISPR/Cas9 mediated genome editing at miR-495 seed sequence. (a) The location and sequence of the sgRNAs, and the template ssOligo for CRISPR/Cas9 editing. miRNA-495 seed sequence and primers specific to the wild-type and mutated alleles were also plotted. (b) Allele-specific primers picked up the mutant form only when both sgRNAs and the ssOligo template were included. (c) Relative number of the mutated alleles to the wild-type alleles after CRISPR/Cas9 mediated genome editing. Quantitative PCR revealed that the relative number of mutated alleles to the wild-type alleles is close to 1/1000. Purified PCR products were serial diluted, and used as templates to construct the standard curve. (d) PAX3 RNA was increased during human MSC muscle differentiation when miR-495 seed sequence was changed to the corresponding sequence of mice. (e) miR-495 suppressed endogneosu PAX3, but not the fusion. RH30 cells were transfected with miR-495 mimics or the control miR-CT. Western blot analysis were performed using FOXO1, PAX3, and GAPDH antibodies (left). Quantification of protein signal intensity was conducted using densitometry, and plotted on the right. *, p < 0.05; **, p < 0.01; ***, p < 0.001.
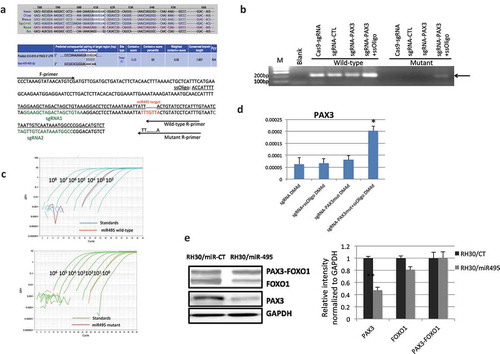