Figures & data
Figure 1. High RapZ concentrations trigger accumulation of processed GlmZ but do not completely turn over full-length GlmZ. Northern analyses addressing abundance of full-length and processed GlmZ in various genetic backgrounds (top panel) and the consequences for GlmS protein levels as detected by immunoblot analyses (panel 3 from top). Strains R1279 (wild type, lane 1), Z45 (ΔglmZ, lane 2), Z95 (ΔglmY, lanes 3–5) and Z37 (ΔrapZ, lanes 6–10) were used, which occasionally harboured the following plasmids: pBR-plac (VC = vector control; lane 4), pYG83 (lane 5), pBGG61 (lanes 7, 8), pBGG164 (lanes 9, 10). The bacteria were grown to exponential phase and harvested for isolation of total RNA and total protein, respectively. 5 μg of the total RNA preparations were subjected to Northern analysis using probes directed against GlmZ (top panel) and 5S rRNA as loading control (second panel from top). Total protein extracts were separated by SDS PAGE and analysed by Western blotting using antisera directed against GlmS (panel 3 from top), ribosomal protein S2 as loading control (panel 4 from top), RapZ (panel 5 from top), and the Strep epitope (panel 6 from top). Non-specific signals are indicated with asterisks. Following blotting, the SDS PAA gel was stained with Coomassie blue to visualize overproduced Strep-RapZ and to provide a further loading control (bottom panel). Arabinose and IPTG were added to the cultures for induction of rapZ expression as indicated.
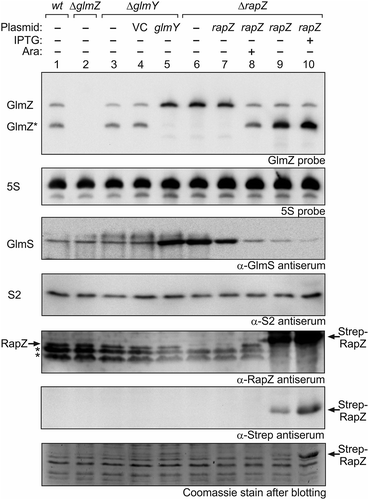
Figure 2. GlmZ* competes efficiently with full-length GlmZ for binding RapZ and inhibits cleavage of full-length GlmZ by RNase E in vitro. (a) EMSA demonstrating that full-length and processed GlmZ can displace each other from complexes with RapZ. α-32P-UTP labelled full-length GlmZ (left) and GlmZ* (right) were pre-incubated with 1500 nM RapZ to achieve complex formation. Subsequently, incremental concentrations of non-labelled GlmZ* (left) and full-length GlmZ (right) were added, respectively. Following an additional incubation step, binding reactions were separated by non-denaturing gel electrophoresis and gels were analysed by phospho-imaging. (b) In vitro cleavage assay demonstrating that GlmZ* inhibits cleavage of full-length GlmZ by RapZ/RNase E. α-32P-UTP labelled full-length GlmZ was incubated alone (‘RNA only’, lane 1) or solely with 25 nM RNase E-NTD (lane 2) or 20 nM RapZ (lane 3), or with both proteins simultaneously (lanes 4–14). In the latter case, various concentrations of non-labelled GlmZ* or GlmY were added as indicated. Following incubation, the reaction products were separated on denaturing PAA gels, which were subsequently analysed by phospho-imaging. Non-specific cleavage products are indicated by asterisks on the right. (c) Left: Northern blot addressing half-life of full-length and processed GlmZ following treatment with rifampicin. Wild type strain R1279 was grown to exponential phase and treated with rifampicin to stop transcription. At indicated times samples were removed for isolation of total RNA, which was analysed by Northern blotting using probes directed against GlmZ (top) and the 5S rRNA (bottom). Right: Semi-logarithmic plots of GlmZ* and full-length GlmZ decay for half-life determination. RNA signal intensities were normalized to 5S signals and plotted semi-logarithmically in percent against time. The graph shows the average values of three independent experiments.
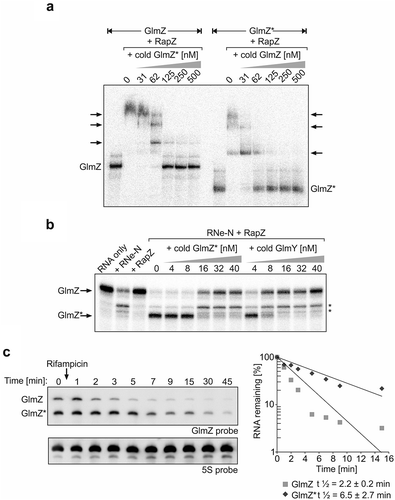
Figure 3. sRNA chimeras mimicking GlmZ* bind RapZ efficiently and counteract cleavage of full-length GlmZ by RNase E in vitro. (a) Schematic representation of the sRNA chimeras used to mimic GlmZ*. Parts derived from GlmZ are depicted in red, the trpA terminator is shown in blue, the glyT tRNA in green and the 6 nt BglII site insertion in chimera 3 is in orange. The structure of full-length GlmZ including location of the processing site (marked with an arrow) is shown for comparison. (b) EMSA demonstrating that RapZ binds GlmZ, GlmZ* and the sRNA chimeras with comparable affinities. α-32P-UTP labelled full-length GlmZ, GlmZ* and sRNA chimeras were incubated with incremental concentrations of RapZ, respectively, and binding reactions were subsequently separated on non-denaturing PAA gels and analysed by phospho-imaging. In case of chimeras 2 and 3, premature transcription termination additionally generated slightly shorter sRNA variants, which were similarly efficient bound as the full-length sRNAs and contributed to additional sRNA/RapZ complexes. It should be noted that complete binding of GlmZ required higher RapZ concentrations as previously reported [Citation30,Citation31], which may be attributed to the individual RapZ protein preparation used. (c) In vitro cleavage assay demonstrating that the various sRNA chimeras impede processing of full-length GlmZ by RapZ/RNase E similarly efficient as GlmZ*. Same assay as in , but as a difference the various non-labelled sRNA chimeras were added.
![Figure 3. sRNA chimeras mimicking GlmZ* bind RapZ efficiently and counteract cleavage of full-length GlmZ by RNase E in vitro. (a) Schematic representation of the sRNA chimeras used to mimic GlmZ*. Parts derived from GlmZ are depicted in red, the trpA terminator is shown in blue, the glyT tRNA in green and the 6 nt BglII site insertion in chimera 3 is in orange. The structure of full-length GlmZ including location of the processing site (marked with an arrow) is shown for comparison. (b) EMSA demonstrating that RapZ binds GlmZ, GlmZ* and the sRNA chimeras with comparable affinities. α-32P-UTP labelled full-length GlmZ, GlmZ* and sRNA chimeras were incubated with incremental concentrations of RapZ, respectively, and binding reactions were subsequently separated on non-denaturing PAA gels and analysed by phospho-imaging. In case of chimeras 2 and 3, premature transcription termination additionally generated slightly shorter sRNA variants, which were similarly efficient bound as the full-length sRNAs and contributed to additional sRNA/RapZ complexes. It should be noted that complete binding of GlmZ required higher RapZ concentrations as previously reported [Citation30,Citation31], which may be attributed to the individual RapZ protein preparation used. (c) In vitro cleavage assay demonstrating that the various sRNA chimeras impede processing of full-length GlmZ by RapZ/RNase E similarly efficient as GlmZ*. Same assay as in Figure 2B, but as a difference the various non-labelled sRNA chimeras were added.](/cms/asset/4ec87297-8c07-474f-a0f6-e3e527b1fb9c/krnb_a_1612693_f0003_c.jpg)
Figure 4. The sRNA chimeras mimicking GlmZ* counteract GlmZ processing in vivo thereby augmenting GlmS levels. (a) Plasmid-driven expression of the sRNA chimeras inhibits processing of endogenously encoded GlmZ. Strains R1279 (wild type, lanes 1–5) and strain Z45 (ΔglmZ, lanes 6–10) harbouring plasmids encoding GlmY (pYG83, lanes 2 and 7), sRNA chimera 1 (pSD164, lanes 3 and 8), chimera 2 (pSD174, lanes 4 and 9), chimera 3 (pSD175, lanes 5 and 10) or the empty plasmid pBR-plac (lanes 1 and 6) were grown in LB to exponential phase for isolation of total RNA. 4 μg total RNA each were analysed by Northern blotting using probes directed against GlmZ (top panel) and 5S rRNA (bottom panel). (b) sRNA chimera 3 counteracts degradation of full-length GlmZ. Northern blot addressing decay of full-length GlmZ in wild type strain R1279 in the presence of the empty plasmid pBR-plac (top panel) or plasmid pSD175 encoding chimera 3 (third panel from top). The transformants were grown to exponential phase and treated with rifampicin to stop transcription. Samples were removed at indicated times for isolation of total RNA, which was analysed by Northern blotting using probes directed against GlmZ and the 5S rRNA. To verify that the slower migrating band detected for transformant R1279/pSD175 corresponds to full-length GlmZ, the total RNA sample obtained before rifampicin addition (time 0) was analysed by Northern blotting (bottom panel) alongside total RNA samples of wild type strain R1279 (lane 1), R1279 carrying the empty plasmid pBR-plac (lane 2) and of the ΔglmZ mutant strain Z45 (lane 3). (c) Expression of the sRNA chimeras increases expression of a glmS’-lacZ reporter fusion, which becomes more pronounced in a ΔglmY mutant strain. Strains Z8 (wild type) and Z884 (ΔglmY) were employed, which carry a glmS’-lacZ fusion integrated into the λattB site on the chromosome. The strains harboured the plasmids described in (a) as indicated. Additionally, transformants carrying plasmid pYG84 overexpressing GlmZ were included for comparison. (d) Effect of the sRNA chimeras on GlmS protein levels. Protein extracts were prepared from the wild type strain R1279 and the ΔglmY mutant strain Z95, which harboured plasmid pBR-plac (empty plasmid, lanes 1 and 6), pYG84 (GlmZ, lanes 2 and 7), pSD164 (chimera 1, lanes 3 and 8), pSD174 (chimera 2, lanes 4 and 9) or pSD175 (chimera 3, lanes 5 and 10). The extracts were subjected to Western blotting using antisera against GlmS (top panel) and S2 protein (bottom panel). (e) Model illustrating feedback regulation of GlmZ processing by processed GlmZ. Tetrameric RapZ (yellow) recruits RNase E (grey) to elicit cleavage of bound full-length GlmZ. Processed GlmZ retains the elements required for interaction with RapZ and sequesters RapZ when accumulating to high levels. This reduces ongoing processing of GlmZ, which is then free to associate with Hfq (green hexamer) and to stimulate glmS expression by base-pairing. This mechanism may absorb fluctuations in RapZ and RNase E availabilities thereby setting a robust basal glmS expression level.
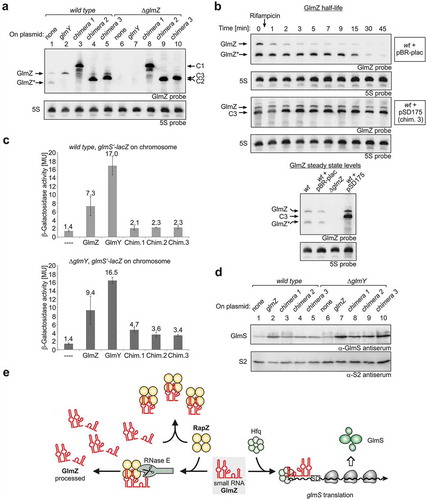
Table 1. Strains and plasmids used in this study.