Figures & data
Figure 1. Mechanisms of transcriptional regulation by lncRNAs. (A). Recruitment of chromatin remodelling complex PRC2 by lncRNAs to induce epigenetic silencing. (B). Binding to DNA methyltransferase DNMT1 and inhibiting its epigenetic modification. (C). Formation of R-loops by binding of lncRNA to the DNA duplex allowing access of the transcription factor (NF-kB) to the promoter of target genes. (D). Recruitment of a transcription factor (TF) by lncRNA to the promoter of the target gene. (E). Suppression of transcription by lncRNA GAS5, which functions as a decoy glucocorticoid response element (GRE) for the glucocorticoid receptor (GR). (F). Binding of DHFR lncRNA to the transcription factor TFIIB to suppress its transcription activity.
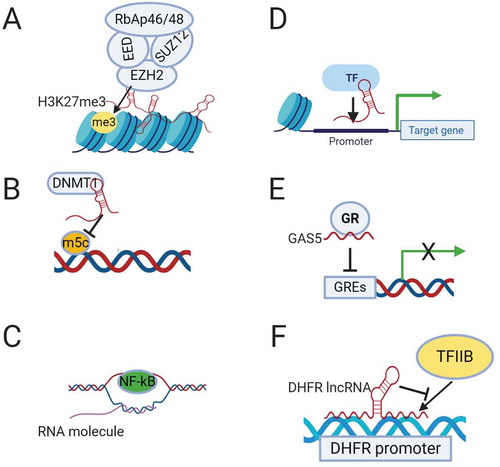
Figure 2. Regulation of splicing by lncRNAs. (A) MALAT1 modulates the localization and phosphorylation of serine arginine rich splicing factors (SR). (B) MALAT1 disrupts the interaction between the splicing factor SFPQ and the proto-oncogene PTBP, releasing PTBP from SFPQ. (C) LINC01133 functions as a decoy for the splicing factor SRSF6, blocking its effect on target mRNAs. (D) SAF NAT forms base-pairing with FAS pre-mRNA to recruit the splicing factor SPF45 in order to induce exon 6 exclusion. (E) ZEB2-AS1 blocks the splicing donor site on ZEB2 mRNA, leading to the inclusion of an IRES that promotes ZEB2 mRNA translation. (F) The lncRNA asFGFR2 recruits polycomb-group proteins and KDM2a to FGFR2 pre-mRNA, masking the binding site of the chromatin splicing complex and causing exon IIIb inclusion.
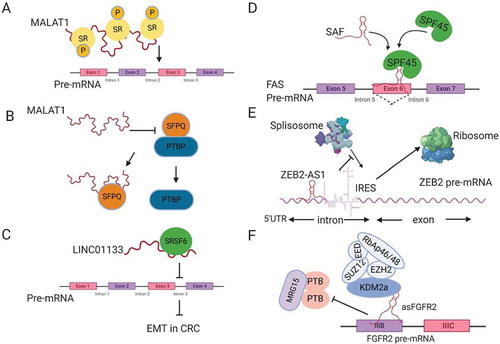
Figure 3. LncRNA regulation of mRNA stability. (A) Linc-ROR mediates binding of hnRNP I to c-Myc mRNA and prevents AUF1 from binding to c-Myc. (B) LncRNA PDCD4-AS1 forms base-pairing with PDCD4 mRNA, blocking HuR-binding site. (C) LncRNA MACC1-AS1 directly binds MACC1 mRNA and stabilizes the mRNA by activating AMPK and inducing lin28 binding to MACC1 mRNA. (D) LncRNA OCC-1 binds HuR RBP and enhances its interaction with E3 ubiquitin ligase β-TrCP1 for proteasomal degradation. (E) LncRNA lnc-ASNR, sequesters AUF1 in the nucleus and reduces its cytoplasmic pool, leading to an increase in the stability of AUF1 target mRNAs. (F) ½ sbsRNA lncRNA forms base-pairing with an Alu element at the 3´UTR of SMD target mRNAs to generate STAU1-binding site (SBS). STAU1 recognizes SBS and binds the ½ sbsRNA/mRNA duplex to initiate SMD.
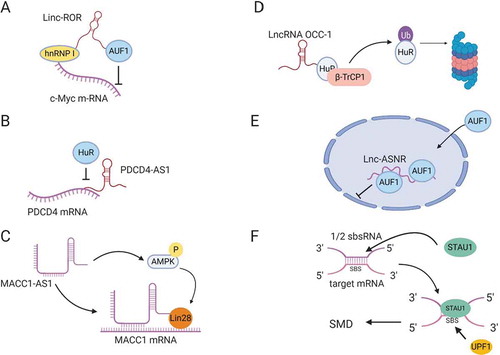
Figure 4. TE-mediated regulation of lncRNA metabolism and function in both cis and trans manners that can influence cancer development. (A) TEs can modulate lncRNA localization and stability by functioning as binding sites for RBP complexes. (B) TEs can function as miRNA binding sites on lncRNAs to control lncRNA stability and may sequester miRNAs from their active binding sites on other transcripts. (C) TEs may function as splice-sites that can be recognized by splicing regulators such as HNRNPC to regulate maturation of pre-lncRNAs. (D) TEs can from PAS to promote APA and formation of short lncRNA transcripts. (E) TEs promote formation of transcription factor binding sites to regulate lncRNA transcription. (F) TEs mediate lncRNA binding to mRNA through partial complementary base pairing in order to promote SMD. (G) TEs mediate antisense lncRNA’s ability to promote translation of sense mRNA. (H) TEs can also mediate lncRNA’s recruitment of chromatin remodelling factors to promoter regions of certain gene loci through formation of dsDNA-RNA triplexes in order to regulate mRNA transcription. (I) TEs embedded in lncRNA can direct RBP localization, aggregation/folding and function.
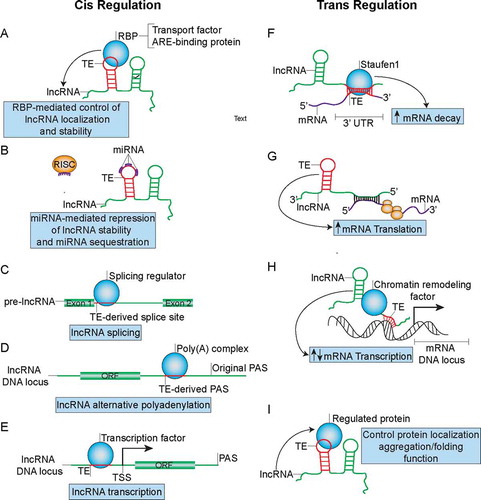
Figure 5. Targeting lncRNAs with ASOs. When administered, ASOs bind to target RNAs with base pair complementarity and can exert various effects. Three mechanisms commonly used in preclinical models and human clinical trial development are shown. These mechanisms include: 1) Targeted degradation of mRNA or lncRNA via recruitment of RNase H, 2) Alternative splicing modification to include or exclude exons, and 3) Causing a steric block that inhibits RNA or protein interactions.
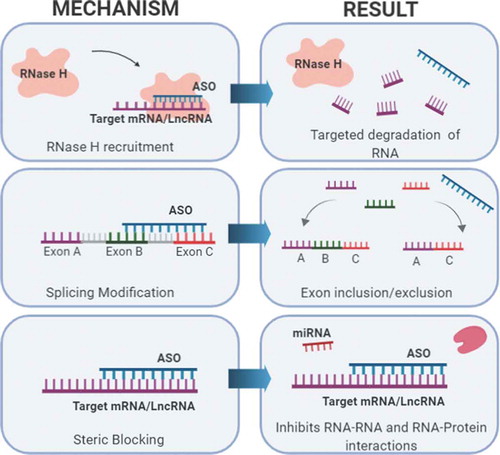