Figures & data
Figure 1. 5′P-seq detected processing sites in the polycistronic pre-rRNA of M. psychrophilus. (a) Schematic shows the 5′P-seq reads and genome location of the polycistronic pre-rRNA processing sites, in which the representative ones are noted in (b) with the same coloured arrows and letters. Arrows in brown and orange indicate the cleavage sites of ribonucleases RNase P and RNase Z. Arrows in red, magenta, and purple point the processing sites produced by unknown endoribonucleases, and arrow in cyan specifies the mature 5′ end of 5S rRNA. Blue arrows indicate the two transcription start sites (TSSs) of the rrn operon detected by dRNA-seq (Fig. S1). (B) RNA secondary structures of the polycistronic pre-rRNA include six helices (A, B, C, E, F, C′) and a processing stem each in pre-16S and pre-23S rRNAs, and two tRNAs and 5S rRNA.
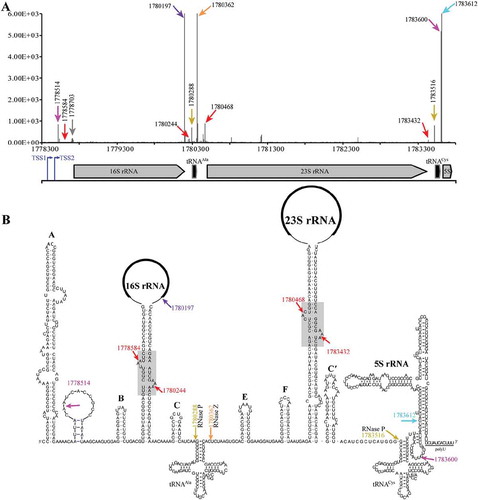
Figure 2. Cleavage at the BHB motifs in the pre-16S and pre-23S rRNA processing stems by EndA. (a) The Mpy-EndA (Mpsy_0954) was overexpressed in E. coli and the purified ~40 kDa protein was detected by SDS-PAGE. (b) Two 42-nt RNA substrates embedded with the 16S and 23S rRNA BHB motifs were synthesized and labelled with 6-FAM at 5′ ends. The cleavage sites of EndA and the predicted product lengths are indicated. (c) Denaturing urea-PAGE analysing the cleavage products of the 16S and 23S rRNA BHB substrates by increased concentrations of Mpy-EndA. The substrates of 42 nt and the cleaved products with expected lengths of 34 nt and 13 nt are indicated by arrows. The gradient protein concentrations are indicated below the grey triangles and 0.15 μM RNA substrate was used. M, low molecular weight marker (10–100 nt).
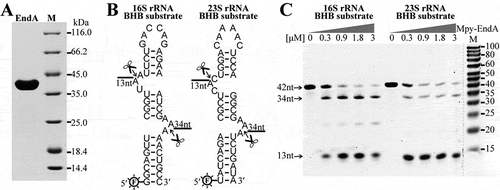
Figure 3. Determination of the presence and percentages of circular pre-rRNA intermediates in total cellular rRNA pool. (a) Schematic shows the predicted circularization coupled with EndA cleaving at BHB motifs (grey shaded boxes, upper panel) to separate pre-16S and pre-23S rRNA from the polycistronic transcript; the spliced products are presumably ligated by RtcB as circular pre-rRNA intermediates (lower panel). Thick black lines indicate mature rRNAs. Thin dark cyan and red lines indicate the precursor sequences from BHB 5′ splice site to the mature 5′ end of 16S or 23S rRNA and that from the mature 3′ end of 16S or 23S rRNA to BHB 3′ splice site, respectively. Same labels are also used in the following figures. (b) Detection of the circular pre-16S and pre-23S rRNA intermediates using ligation-omitted cRT-PCR as described in Materials and methods. The PCR primer pairs are shown in (A), the nested PCR products on cDNA templates that are reverse-transcribed from total RNA with (+) or without (−) T4 RNA ligase pretreatment were detected on a 2% agarose gel (upper panel). Longer and shorter arrows specify the products of the first and second round of PCR, respectively. The lower panels show the DNA sequencing results of the PCR products with an inverted ligation between the 3′ half and 5′ half of 16S and 23S rRNA BHBs. (c–d) Northern blot detected the circular pre-16S (c) and pre-23S rRNA intermediate (d) (left panel) and total cellular 16S or 23S rRNA (right panel) using different probes that spanned the junction region or targeted the mature ends of rRNAs. Locations of the probes are shown in (A). Total RNA contents used are indicated at the top of panel. M1 and M2 indicated ssRNA size markers. (E–F) qRT-PCR determined the percentages of circular pre-rRNA intermediates in the total pool using primers matching the precursor and mature sequences. Percentages of the circular pre-rRNA intermediates in the total were determined at the early, middle, late and stationary cultures. All of the data were calculated from three independent measurements and standard deviations are shown.
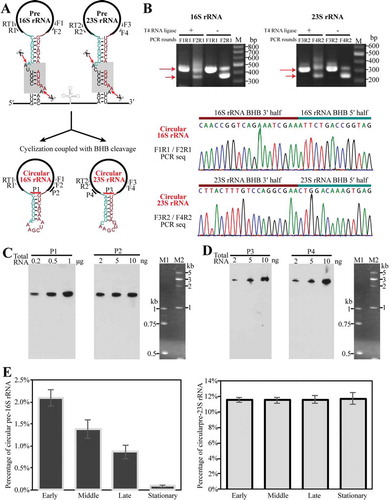
Figure 4. The endoribonuclease Nob1 mediates the linearization of circular pre-16S rRNA intermediate. (a) 3′-RACE determined the mature 3′ end of 16S rRNA. A diagram shows the circular pre-16S rRNA intermediate (left upper panel) with the precursor sequence in the same colour as in . Using a procedure depicted in the upper right panel, the nested PCR products of 3′-RACE were detected by agarose gel electrophoresis and sequenced (lower panel). (b–d) The endoribonucleolytic activity of Mpy-Nob1 was assayed on the RNA substrates of a linear RNA L80 (b), a circular-like RNA SL37 (c), and an actual circular RNA C251 (d). Diagrams display the three RNA substrates as described in Materials and methods. Incubation of gradient concentrations of Mpy-Nob1 as indicated with 0.2 μM L80 (B) and 0.2 μM SL37 substrates (C) respectively and the cleavage products (black arrows) were run on 10% urea-PAGE, while C251 (black circle, 3 nM) and the cleavage products were analysed on a 6% urea-PAGE with L251 substrate as control (D, lane L, black line). The D6N mutant was included in the three substrate cleavage assays. M, low molecular weight marker (10–100 nt). (e) Circular pre-16S rRNA in total RNA was linearized by Nob1. Total cellular RNA was incubated with the indicated concentrations of Mpy-Nob1. After incubation for 30 min, ligation-omitted cRT-PCR was performed using the same approach as in . Agarose gel displays the PCR products of circular pre-16S and pre-23S rRNAs. M in (A) and (E), 100 bp DNA ladder marker showing the migration of PCR products.
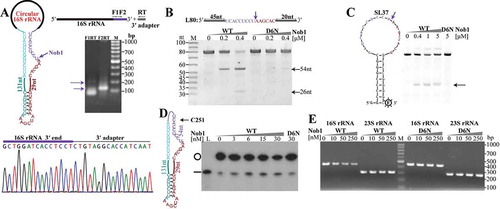
Figure 5. Linearization of the circular pre-23S rRNA intermediate followed by 3′-5′ exoribonucleolytic trimming. (a) Primer extension detected the major processing sites upstream of 23S rRNA. Cyan arrows indicate the two mature 5′ ends of 23S rRNA, and the upstream major processing sites are indicated by the same coloured arrows shown in . 1 h and 3 h at gel top indicate autographing times. (b) 3′-RACE assayed the 3′-5′ exoribonucleolytic processing trace in pre-23S rRNA maturation. A diagram of the circular pre-23S rRNA shows the complete precursor sequence (left upper panel) with the featured RNA fragments coloured same as in . An endoribonucleolytic processing event (dark red arrow) was predicted to linearize the circular pre-23S rRNA. 3′-RACE was performed as depicted in the right upper panel and the products were sequenced. The nested PCR products in 3′-RACE were analysed on 2% agarose gel (right upper panel) and the DNA sequencing results of nt 1780489 (right lower panel) are shown. The remaining six representative 3′ ends (*2 to *7) captured by 3′-RACE are shown as a diagram (lower panel), and the corresponding sites are indicated in the sequence at the upper panel. Numbers in parenthesis indicate the sequenced clones.
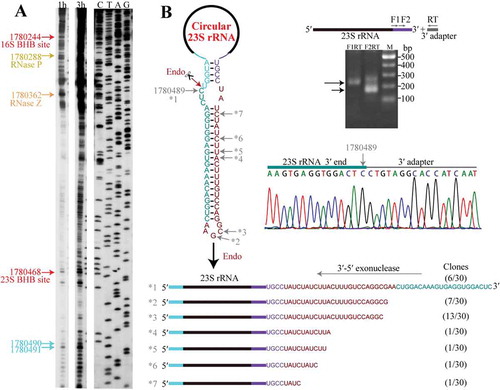
Figure 6. 5′-3′ exoribonucleolytic processing finalizes the 16S rRNA maturation. (a) cRT-PCR determined the mature 5′ and 3′ ends of 16S rRNA as depicted in upper left panel. The nested PCR products were amplified from cDNA templates generated from a reverse-transcription of the total RNA that were pretreated with (+) or without (−) T4 RNA ligase and analysed on an agarose gel (right upper panel) and sequenced (lower panel). Nucleotide 1778722 points the junction of 3′ and 5′ ends of 16S rRNA. (b) Primer extension analysis showing the processing sites at 5′ end of 16S rRNA. Two transcription start sites (TSS1 and TSS2, blue arrows), an endoribonucleolytic site (magenta arrow), 5′ splice site generated by EndA (red arrow) and the mature 5′ end of 16S rRNA (cyan arrow) are pointed. (c) A series of 1-nt 5′P-seq reads between the mature 5′ end (nt 1778722) and upstream nt 1778699 of 16S rRNA exposes a 5′-3′ exoribonucleolytic pattern.
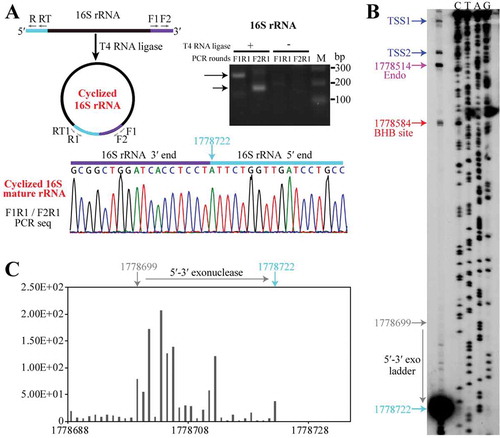
Figure 7. The conserved rRNA maturation modes in archaea. By searching the available archaeal genomes in KEGG database, key elements involved in pre-rRNA processing and maturation were analysed in 30 representative species affiliating to the four superphyla of archaea. These include three organization patterns of rRNA operons (a), 16S and 23S rRNA BHB motifs (b), 16S 3′ end sequences cleaved by Nob1 (c), three maturation modes of 5S rRNA (d), and the presence of EndA, RtcB and Nob1 (e). The grey shadowed BHB motif in (B) consists of two bulges (red spheres) and an in-between helix (blue spheres). A conserved 3′ end sequence of 16S rRNA noted as anti-SD in (C) is found in almost all of the investigated archaea, and perfectly complementary to the M. psychrophilus SD sequence (black dot line framed). Three maturation modes of 5S rRNA are summarized in the 30 investigated archaeal species (d,e): (i) transcribed as matured ‘leaderless’ 5S rRNA prevalent in TACK, Asgard and DPANN, (ii) RNase Z-dependent maturation on a tRNA-like structure widely distributed in haloarchaea, and (iii) ‘endo+exo’ mode employed in methanoarchaea. A representative phylogenetic tree (e) is constructed on the homology analysis of the protein concatenation of EndA and Nob1 from the 30 investigated archaeal species. The tree was constructed using MEGA7.0, and the bar represents 10% sequence difference. E, Euryarchaeota (green background); T, Thaumarchaeota (brick red background); A, Aigarchaeota (blue background); C, Crenarchaeota (pink background); K, Korarchaeota (magenta background); N/A, Nanoarchaeota and ARMAN (yellow background). Distributions of the key elements involved in rRNA maturation are shown at the right side. Filled and empty symbols represent the presence and absence of items in corresponding archaeal species. Grey filled squares indicate incomplete or inaccurate 16S or 23S rRNA sequences that do not allow a prediction of BHB motif, and NA means no available 16S or 23S rRNA sequence in the database. Grey shaded letters in 3′ end of 16S rRNA indicate mutated sites by comparing with the conserved mature 3′ end sequence shown in (C).
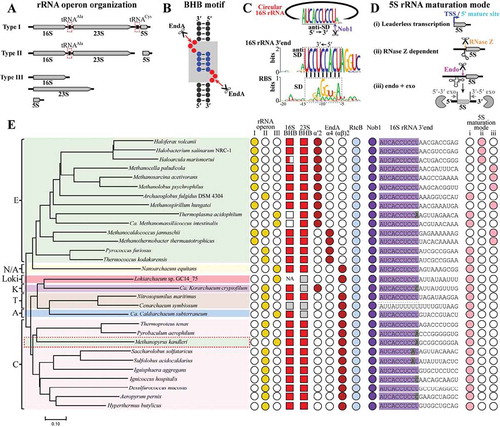
Figure 8. The pre-rRNA processing and maturation mode in M. psychrophilus. The rRNA operon (16S-tRNAAla-23S-tRNACys-5S) is transcribed as a polycistronic RNA from two transcription start sites (TSS1 and TSS2). A co-transcriptional endoribonucleolytic cleavage (Endo) at 5′ ETS that removes helix A initiates the processing (Step I). Following pre-rRNA transcription and folding, rRNAs and tRNAs are segmented (Step II) by the corresponding endoribonucleases. Splicing endonuclease EndA cleaves at BHB motifs buried in the processing stems to excise pre-16S and pre-23S rRNAs and RNA ligase RtcB catalyzes the coupled circularization. Cleavages of RNase P and RNase Z release tRNAAla, while cleavages of RNase P and an unknown endoribonuclease separate tRNACys and 5S rRNA. The circular pre-16S and pre-23S rRNA intermediates are linearized (Step III). Nob1 linearizes the circular 16S rRNA at the mature 3′ end and generates a long 5′ extension. In contrast, an unknown endoribonuclease linearizes the circular 23S rRNA at the mature 5′ end and generates a short 3′ extension. Finally, linearized pre-16S, 23S, and 5S rRNAs are trimmed through different exoribonucleolytic pathways (Step IV). The 5′ and 3′ extensions of pre-16S and pre-23S rRNAs are finally matured by 5′-3′ and 3′-5′ exoribonucleolytic trimming, respectively, while exoribonucleolytic trimming of both ends are involved in 5S rRNA maturation. The scissor and Pacman icons represent an endoribonuclease and exoribonuclease, respectively. Dark cyan and red lines at pre-16S and pre-23S rRNAs show the precursor regions in circular intermediates. Magenta dotted line frames a postulated pre-5S rRNA maturation mode by a combination of endoribonucleolysis and exoribonucleolysis.
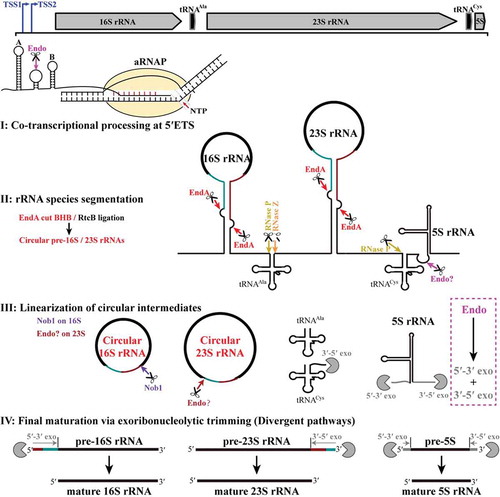