Figures & data
Figure 1. The duplex unwinding activity of mpy-RNase J on hairpin RNAs. (A) Kinetic analysis of mpy-RNase J degrading 3′-radiolabeled hairpin RNAs (Gx) that have a common 9-bp duplex but linking variable nt (suffixed numbers) of 5′ single-stranded overhangs. Nuclease assays were conducted as described in the Materials and Methods, and the radiolabeled Gx substrates with indicated sizes were used as the migration ladders of cleavage products. The initial reaction rates of plotting the decrease of RNA substrates are shown as histograms at right. (B) Kinetic analysis of degrading the 3′-radiolabeled 8-nt 5′ ss overhang RNAs (Dx) carrying duplex lengths of 5, 7, 9, and 11 bp indicated as suffix of D. Nuclease assays were conducted as in (A) except using five-fold higher protein concentrations. Reaction products were analysed on 10% PAGE gels (left panel). Arrows indicate the three types of major products noted as ‘largest, medium, and final’ according to the migrations. The final products, CMP and pCp, were also determined with thin layer chromatography (TLC) plates in parallel (right upper panels). Percentages of the three major products released at 30 min are shown as a histogram in the right lower panel.
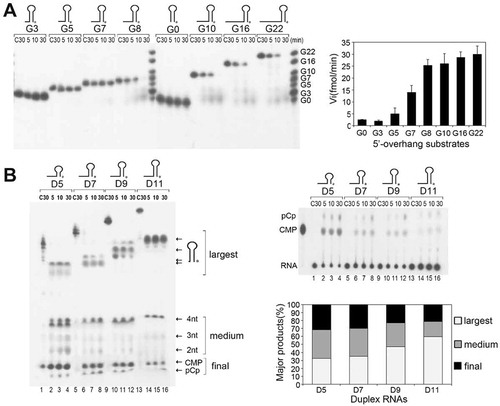
Figure 2. The duplex unwinding activity of mpy-RNase J on hybrid RNA-DNA substrates (Hx). (A) Kinetic analysis was assayed using the 3′-end 6-FAM labelled Hx substrates that share a common 20-nt 3′-labelled ssRNA and hybridized with varying-length complementary DNA strands. Suffixed numbers refer to the 5′ ss overhang lengths. 3′-labelled ssRNAs with indicated sizes were used as ladders. A ssRNA S20 was included in the assays. (B) Degradation of 3′-labelled 10 nt 5′ ss overhang RNAs (dx). Suffixed numbers refer to the duplex (d) lengths of 20, 30, and 40 bp. Nuclease assays were conducted as in (A), but with gradient concentrations of mpy-RNase J as indicated. The cleavage products were analysed on 10% PAGE gels (left panel). The final products, UMP-6FAM and the bottom 6FAM are indicated.
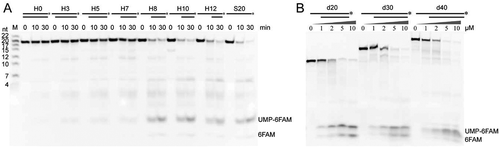
Figure 3. The rA6-mpy-RNase J-S247A structure suggests a hydrolysis-coupled and steric occlusion-based duplex unwinding mechanism. (A) Cartoon overall structure of rA6-mpy-RNase J-S247A. A 6 nt poly(A) RNA within the channel is shown in sticks and surrounded by a ligand-omitted 1Fo-Fc map contoured at 3o’. (B) Interactions of rA6 RNA with mpy-RNase J. (C) A proposed steric barrier comprising two unique archaeal loops in addition to helices α5 and α9. Carbon, oxygen, and nitrogen atoms are indicated in red, grey, and blue, respectively. Archaeal loop I, loop II, helix α5, and helix α9 are shown in green, cyan, yellow, and red, respectively. Key residues in the predicted elements that constitute the steric barrier and RNA interactions are depicted as sticks.
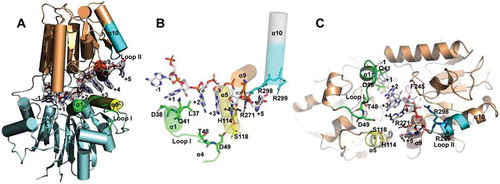
Figure 4. The coupled duplex unwinding and exoribonuclease activities of mpy-RNase J. Residue substitution mutants were constructed in the key elements involving in the exoribonucleolytic cleavage and processivity, which include the catalytic active site, hydrogen-bond network flanking the catalytic centre, and the 5ʹ monophosphorylated (5ʹP) pocket. The 5ʹ end exoribonucleolytic cleavage activity (A), the 5ʹ to 3ʹ exoribonucleolytic processivity (B), and the duplex unwinding activity (C) of mpy-RNase J and its variants were compared on the 5ʹ end-labelled S10, 3ʹ end-labelled S20, and 3ʹ end-labelled H8 RNA substrates. 10 μM of mpy-RNase J and its mutants were used in the nuclease assays. Cleavage products were analysed using PAGE gel, and migrations of ssRNA markers with indicated lengths are labelled on the left of the gels.
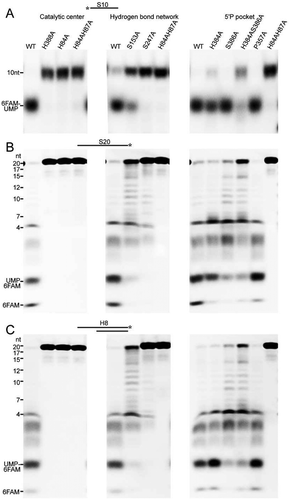
Figure 5. Roles of base stacking at Arg271 and the proposed steric barrier involved in RNA translocation and duplex unwinding of mpy-RNase J. (A) Contribution of stacking interactions between bases +2 to +5 and Arg271 to RNA translocation in the processive 5ʹ to 3ʹ exoribonucleolysis. The 5ʹ to 3ʹ exoribonucleolytic processivity of mpy-RNase J was compared using 3ʹ-labelled S19 and its mutant S19-M4 that carries four consecutive base lacking nucleotides. A mixture of ssRNAs with indicated lengths was used as size markers at the gel left (lane M). A synthetic 3ʹ-labelled S11-M4 shown at right was also used as size marker for the smallest degradation intermediate. The schematic in the right panel shows the five degradation intermediates from S19-M4. (B, C) Effects of the proposed steric barrier mutations on duplex unwinding activity of mpy-RNase J. Ten key residues in the archaeal loops I and II and the helices α5 and α9 that constitute the proposed steric barrier were mutated, and the duplex unwinding activities of mpy-RNase J and the mutants were determined by using the hairpin G8 (B) and hybrid H8 RNA (C) substrates. Stalled degradation intermediates at duplex structures are indicated by arrows. 10 μM of mpy-RNase J and its mutants were used in the nuclease assays, and CK indicates the assay without enzyme. The cleavage products were analysed on PAGE gel, and migrations of ssRNA markers with the indicated sizes are shown on the left.
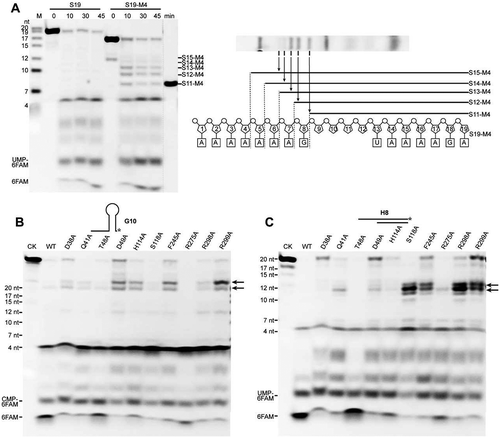
Figure 6. Duplex unwinding activity of bacterial sco-RNase J determined using hybrid RNA substrates (Hx). (A) Using the same Hx RNAs as in , degradation kinetics of sco-RNase J were assayed in the same way as those of mpy-RNase J. (B) Degradation of the 3′-labelled 10 nt 5′ ss overhang dx RNAs by sco-RNase J. The same dx RNAs in and 10 μM of sco-RNase J protein were used for nuclease assays. The reaction products were analysed by 10% PAGE gels, and the final products, UMP-6FAM and the bottom 6FAM are indicated.
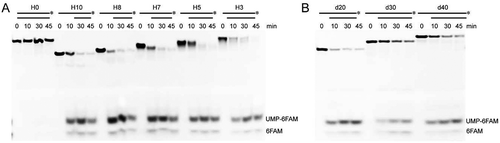
Figure 7. The degradation activity of mpy-RNase J on highly structured mRNAs. Five highly structured mRNAs were used as substrates, including four 5ʹ UTR RNAs from Mpsy_3111, Mpsy_1580, Mspy_1767, and Mpsy_2714 of M. psychrophilus, and a REP-structured RNA from the E. coli malEF intergenic region. RNA lengths, secondary structures, and folding ΔG values are shown in the left panels. Nuclease assays were conducted using the indicated concentrations of mpy-RNase J and the reaction conditions described in the Materials and Methods. Cleavage products were analysed by 10% PAGE gels. Migrations of the ssRNA markers with indicated length are shown at the left of the gels.
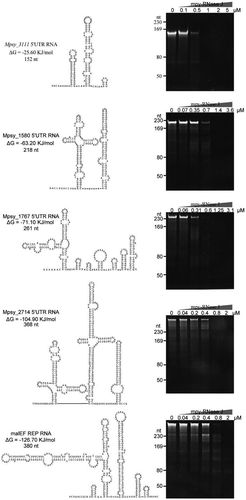
Figure 8. The proposed exoribonucleolysis-coupled and steric occlusion-based duplex unwinding mechanism of archaeal RNase J. (A) To initiate duplex RNA degradation, mpy-RNase J requires a ≥ 7 nt 5′ single-stranded overhang, which is long enough to reach the catalytic site at the RNA channel end for initiating exoribonucleolysis. (B) Following each 5′ end cleavage, the 5′p (n-1) RNA is translocated by advancing 1 nt in each exoribonucleolytic cycle using the hydrolysis released energy. (C) Along with the forward RNA translocation, a steric barrier at the RNA channel entrance, which is constituted by the archaeal loops I and II and helices α5 and α9, only allows the passage of single-stranded RNAs but sterically restricts and unwinds double-stranded RNAs. (D) The synergistic action of the forward RNA translocation within the channel and the steric occlusion at the channel entrance unwinds the duplex regions, thereby enabling mpy-RNase J to degrade the structured RNAs.
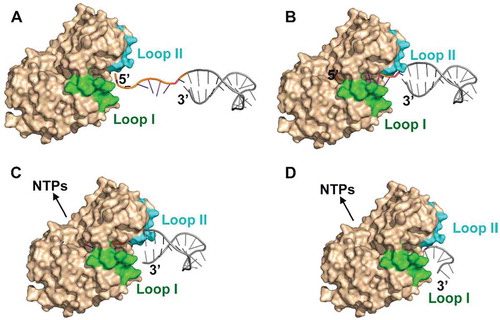