Figures & data
Figure 1. Design of a mistranslation sensitive fluorescent protein. To detect mistranslation events, a mutant tRNASer with a proline anticodon is co-expressed with a fluorescently inactive mCherry Ser151Pro mutant (a). If no mistranslation event occurs at position 151, then the mCherry is accurately made and does not fluoresce. A mistranslation event at position 151 that incorporates serine instead of proline will restore fluorescent activity. Ser151 interacts with the mCherry chromophore (Met71-Tyr72-Gly73, PDB code 2H5Q [Citation31]) via a hydrogen bond (b). We anticipated that mutation at Ser151 would interfere with the chromophore and disrupt mCherry fluorescence.
![Figure 1. Design of a mistranslation sensitive fluorescent protein. To detect mistranslation events, a mutant tRNASer with a proline anticodon is co-expressed with a fluorescently inactive mCherry Ser151Pro mutant (a). If no mistranslation event occurs at position 151, then the mCherry is accurately made and does not fluoresce. A mistranslation event at position 151 that incorporates serine instead of proline will restore fluorescent activity. Ser151 interacts with the mCherry chromophore (Met71-Tyr72-Gly73, PDB code 2H5Q [Citation31]) via a hydrogen bond (b). We anticipated that mutation at Ser151 would interfere with the chromophore and disrupt mCherry fluorescence.](/cms/asset/c2f26d13-ff05-44d6-9b0c-eda7816d2f11/krnb_a_2015173_f0001_oc.jpg)
Figure 2. Growth on solid media of wild-type or mistranslating E. coli expressing mCherry variants. E. coli BL21 cells were transformed with plasmids expressing either wild-type mCherry with a Ser151 AGC codon or mutant mCherry with a Pro151 CCA codon. Each strain was co-transformed with a second plasmid bearing either wild-type tRNASer, derived from the GCT 1–1 tRNASer gene (a) or the GGA 1–1 tRNASer gene (b), or a mutant version of each tRNASer gene that has a UGG proline-decoding anticodon. mCherry with a Pro151 codon co-expressed with either of the tRNASerUGG variants demonstrated fluorescence rescue resulting from mistranslation. For the negative controls, a wild-type tRNASer was co-expressed with a mutant mCherry Pro151 that did not fluoresce above the background. Cultures from three biological replicates were aliquoted in serial dilution as indicated in Methods.
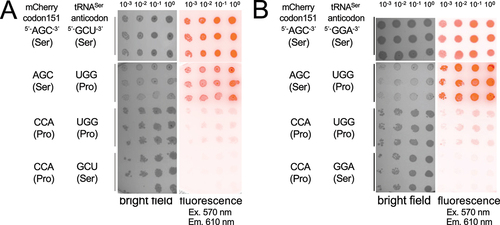
Figure 3. Growth of E. coli strains expressing wild-type or mistranslating tRNAs. Using a 96-well microplate, cell growth (A600) was recorded over a 16-hour time course for (a) E. coli cells co-expressing mCherry with tRNASerGCU (blue circles), mCherry with mutant tRNASerUGG (red triangles), mCherry Ser151Pro with tRNASerUGG (Orange triangles), or mCherry Ser151Pro with wild-type tRNASerGCU (grey circles). Cell growth (c) is also shown for E. coli cells expressing mCherry with tRNASerGGA (blue circles), mCherry with a corresponding mutant tRNASerUGG (red triangles), Ser151Pro with mutant tRNASerUGG (Orange triangles), Ser151Pro with tRNASerGGA (grey circles). At each time point, fluorescence (ex 570 nm, em 610 nm) intensity was also recorded (b, d). The inset panels (b, d) show a zoomed in view of the fluorescence recovery due to serine mis-incorporation at Pro151.
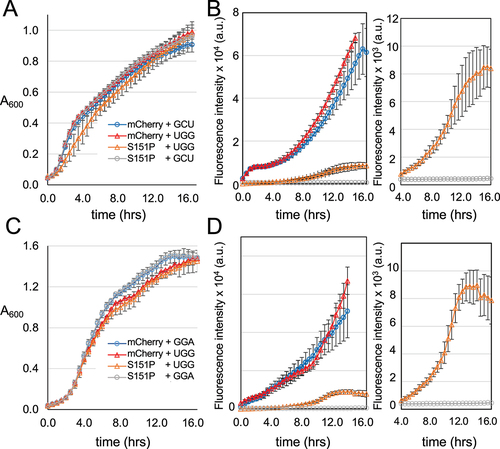
Table 1. Ser mis-incorporation levels in cells and purified mCherry proteins
Figure 4. Quantification of mistranslation level and rate in live E. coli cells. Data from cell growth and mCherry fluorescence in microplates () were analysed to compare the maximal fluorescence per cell observed in each of the indicated mCherry producing strain (a, b). The rate of fluorescence increase per cell (c, d) was calculated by measuring the slope of the linear phase of fluorescent mCherry production per cell (,d)). Data are shown for the tRNASerGCU derived variants (a, c) and for the tRNASerGGA derived variants (b, d). Error bars represent ± 1 standard deviation of three biological replicates and three technical replicates. Statistical significance is based on ANOVA single factor analysis (n. s. – not significant, ** p < 0.01).
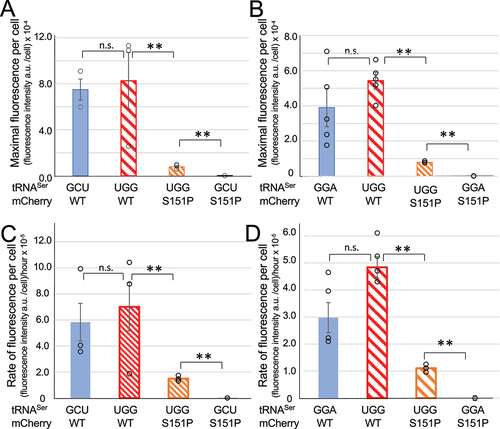
Figure 5. Fluorescent measurements of purified mCherry variants. mCherry proteins variants were purified and fluorescence (ex 570 nm, em 610 nm) of each serial dilution (a, b) was measured. Based on linear regression of the data (A,B dotted lines), the slope is the specific fluorescence or fluorescence intensity per amount of mCherry protein (c, d). The wild-type or Ser151Pro mCherry variants were produced with co-expression of either the (a) tRNASerGCU or a UGG anticodon mutant or with the (b) tRNASerGGA or a UGG anticodon mutant. According to specific fluorescence, the fluorescence recovery or serine mis-incorporation level is (c) 4.1% for the tRNASerGCU derived proline decoding mutant and (d) 9.7% for tRNASerGGA derived mutant. Statistical significance is based on ANOVA single factor analysis (n. s. – not significant, ** p < 0.01).
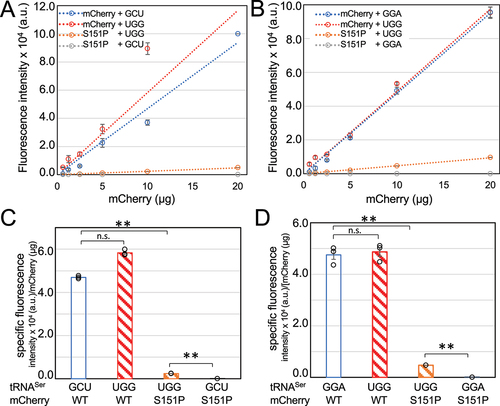
Figure 6. Tandem mass spectrometry analysis reveals Ser mis-incorporation in mistranslating cells at Pro151. MS/MS coverage of purified and trypsin digested mCherry variants is represented as a schematic with each blue bar representing an identified peptide. MS/MS spectra for representative peptide hits are shown (right). Cells co-expressing wild-type mCherry with a mutant tRNASerUGG (a) showed only Ser151 as expected. Cells co-expressing the mutant mCherry with a wild-type tRNASer (b) showed only Pro151. Mistranslation was readily detected in cells expressing both mutant mCherry and mutant tRNA (c) where multiple high-quality spectra were identified for peptides containing either Pro151 or Ser151.
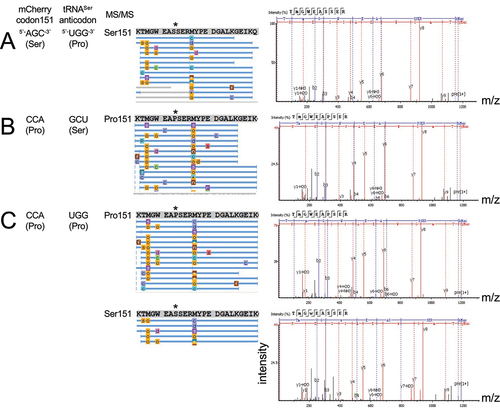
Table 2. Two-sample KS test results from comparing RMSD distributions of mCherry variants
Figure 7. Conformational sampling explains the mechanism of fluorescence inhibition and rescue. A set of 1,000 plausible conformations of the entire mCherry precursor protein were generated in Rosetta for WT, Ala150Gly, Ser151Pro and Ser151Phe mutants. (a) The distribution in chromophore precursor position was compared to that of the mature chromophore in the 2H5Q mCherry crystal structure [Citation31]. (b) The position distribution for each mutant is represented as RMSD values (x-axis) of the chromophore precursor atoms compared to the initial starting model. The Rosetta Score (y-axis), a metric used to estimate relative conformational stability, is also shown for each generated model.
![Figure 7. Conformational sampling explains the mechanism of fluorescence inhibition and rescue. A set of 1,000 plausible conformations of the entire mCherry precursor protein were generated in Rosetta for WT, Ala150Gly, Ser151Pro and Ser151Phe mutants. (a) The distribution in chromophore precursor position was compared to that of the mature chromophore in the 2H5Q mCherry crystal structure [Citation31]. (b) The position distribution for each mutant is represented as RMSD values (x-axis) of the chromophore precursor atoms compared to the initial starting model. The Rosetta Score (y-axis), a metric used to estimate relative conformational stability, is also shown for each generated model.](/cms/asset/87d9d4c2-e861-4c3c-9291-19a5d3321e34/krnb_a_2015173_f0007_oc.jpg)