Figures & data
Figure 1. (A) Challenges and strategies for developing efficient and safe ssONs in the clinic. (B) Challenges and strategies for the development of efficient and safe siRNAs. (C) RNA-centric killing of a bacterium of interest can be achieved by delivering a short antisense oligomer (ASO), here a peptide nucleic acid (PNA), to sequester the 5’ region of the mRNA of an essential gene. Such ASOs are coupled to small uptake or cell-penetrating peptides that carry them inside the bacteria. The mechanisms of transport into the bacteria and whether peptide and ASO remain attached to each other, or cleaved after entry, are not completely understood. (D) Suggested mechanism for small activating RNAs. 1) The double stranded saRNA is taken up into the cell by endocytosis. 2) Then, the double stranded saRNA is loaded into an AGO2 protein. 3) The passenger strand of the saRNA is cleaved and discarded in the cytoplasm as an active saRNA–AGO2 complex is formed. 4) The AGO2 bound saRNA is actively transported into the nucleus. 5) The active saRNA–AGO2 complex binds at the promoter region of the gene sequences (complementary DNA or noncoding RNA transcripts) and associates with the RNA helicase RHA and the RNA polymerase-associated protein CTR9. The complex subsequently associates with RNA polymerase II and activates transcription of the targeted gene. 6) The nascent RNA is produced and exported into the cytoplasm to be translated.
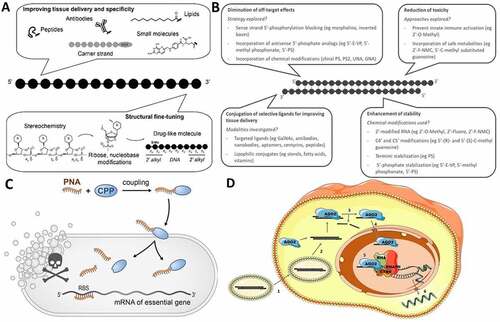
Figure 2. Nucleic acid nanotechnology. (A) Programmable assembly of DNA strands into a 4-way junction with single-stranded toehold regions that promote assembly into higher order structures [Citation128]. (B) Assembly of higher order structures using the programmable base pairing alphabet of DNA. On the left, representation of the Mona Lisa using fractal assemblies of DNA origami [Citation130]. On the right, 3D DNA rabbit assembled from DNA polyhedral meshes [Citation131]. (C) Wireframe minimal 3D NA acid nanostructures in the shape of a cube, a tetrahedron and various prismatic structures [Citation128,Citation141]. (D) Spherical nucleic acids with and amenable cores for nucleic acid functionalization [Citation145]. (E) Functionalization of a L-DNA tetrahedron with siRNAs. This construct was found to accumulate in the kidneys and mediate gene knockdown in vivo [Citation149,Citation150]. (F) SNAs as cancer vaccines. The nanoscale organization of the antigen (green) and adjuvant (blue) determines the efficacy of the overall construct [Citation147].
![Figure 2. Nucleic acid nanotechnology. (A) Programmable assembly of DNA strands into a 4-way junction with single-stranded toehold regions that promote assembly into higher order structures [Citation128]. (B) Assembly of higher order structures using the programmable base pairing alphabet of DNA. On the left, representation of the Mona Lisa using fractal assemblies of DNA origami [Citation130]. On the right, 3D DNA rabbit assembled from DNA polyhedral meshes [Citation131]. (C) Wireframe minimal 3D NA acid nanostructures in the shape of a cube, a tetrahedron and various prismatic structures [Citation128,Citation141]. (D) Spherical nucleic acids with and amenable cores for nucleic acid functionalization [Citation145]. (E) Functionalization of a L-DNA tetrahedron with siRNAs. This construct was found to accumulate in the kidneys and mediate gene knockdown in vivo [Citation149,Citation150]. (F) SNAs as cancer vaccines. The nanoscale organization of the antigen (green) and adjuvant (blue) determines the efficacy of the overall construct [Citation147].](/cms/asset/d39aaf61-af73-4f96-8f7a-28a7053f3556/krnb_a_2027150_f0002_oc.jpg)
Figure 3. (A) Phylogenetic tree depicting the branching of the phi29-like phage genus into groups I, II, and III. Adapted from [Citation176]. (B) Cryo-electron microscopy reconstruction of the phi29 phage packaging motor. The prohead is grey. The multimeric motor components are labelled: connector (cyan), pRNA (magenta), and ATPase (purple). Adapted with permission from [Citation178]. (C) Primary and secondary structure of the phi29 pRNA. Helices and kissing loops are labelled. The three-way junction (3WJ) is highlighted in grey. Lines are Watson-Crick base pairs. Dots are wobble pairs. Adapted from [Citation181]. (D) Dimer of pRNA chimeras. A CD4-binding aptamer is teal, a survivin-targeting siRNA is magenta, and pRNA scaffolds are black. Intermolecular kissing loop interactions are indicated with dashed lines. Adapted from [Citation190]. (E) The pRNA 3WJ assembles from three component strands: 3WJa (green), 3WJb (Orange), and 3WJc (blue). Adapted from [Citation194]. (F) A 3WJ (black) is functionalized with a luciferase-targeting siRNA (purple), folic acid (FA; yellow square), and Alexa Fluor 647 (AF647; red circle). Adapted from [Citation196].
![Figure 3. (A) Phylogenetic tree depicting the branching of the phi29-like phage genus into groups I, II, and III. Adapted from [Citation176]. (B) Cryo-electron microscopy reconstruction of the phi29 phage packaging motor. The prohead is grey. The multimeric motor components are labelled: connector (cyan), pRNA (magenta), and ATPase (purple). Adapted with permission from [Citation178]. (C) Primary and secondary structure of the phi29 pRNA. Helices and kissing loops are labelled. The three-way junction (3WJ) is highlighted in grey. Lines are Watson-Crick base pairs. Dots are wobble pairs. Adapted from [Citation181]. (D) Dimer of pRNA chimeras. A CD4-binding aptamer is teal, a survivin-targeting siRNA is magenta, and pRNA scaffolds are black. Intermolecular kissing loop interactions are indicated with dashed lines. Adapted from [Citation190]. (E) The pRNA 3WJ assembles from three component strands: 3WJa (green), 3WJb (Orange), and 3WJc (blue). Adapted from [Citation194]. (F) A 3WJ (black) is functionalized with a luciferase-targeting siRNA (purple), folic acid (FA; yellow square), and Alexa Fluor 647 (AF647; red circle). Adapted from [Citation196].](/cms/asset/1b766065-d2e8-4187-adc9-7905f0551671/krnb_a_2027150_f0003_oc.jpg)
Figure 4. (A) Schematic presentation of the foreseen action of oligonucleotide-based artificial ribonucleases in a therapeutic setting: 1) Cell entry 2) Sequence-dependent hybridization to the RNA target by Watson-Crick base pairing 3) Cleavage of one or several phosphodiester linkages in the RNA target 4) Release of cleaved RNA fragments 5) Finding the next target to repeat the cycle and give substrate turnover. (B) Mechanism of action of RNA‐PROTACs binding RBPs and directing them to degradation. Structure of Lin28 zinc finger domain binding to its consensus sequence AGGAGAU, which was used as ligand for the PROTAC. (Adapted from Ghidini et al [Citation228].) (C) Proposed mode of action of an antibody−PROTAC conjugate, resulting in HER2-dependent protein degradation and its overall structure. (Adapted from Maneiro et al [Citation231].).
![Figure 4. (A) Schematic presentation of the foreseen action of oligonucleotide-based artificial ribonucleases in a therapeutic setting: 1) Cell entry 2) Sequence-dependent hybridization to the RNA target by Watson-Crick base pairing 3) Cleavage of one or several phosphodiester linkages in the RNA target 4) Release of cleaved RNA fragments 5) Finding the next target to repeat the cycle and give substrate turnover. (B) Mechanism of action of RNA‐PROTACs binding RBPs and directing them to degradation. Structure of Lin28 zinc finger domain binding to its consensus sequence AGGAGAU, which was used as ligand for the PROTAC. (Adapted from Ghidini et al [Citation228].) (C) Proposed mode of action of an antibody−PROTAC conjugate, resulting in HER2-dependent protein degradation and its overall structure. (Adapted from Maneiro et al [Citation231].).](/cms/asset/d82d0399-0135-43d5-b6b4-bcfededfe367/krnb_a_2027150_f0004_oc.jpg)