Figures & data
Figure 1. (A) Depiction of the secondary structure of xrRNABNYVV-fs, as determined in our previous study [Citation31], with some changes as described in the main text. Nucleotides are numbered from the first residue in the hp1 stem, and coloured according to the hypothesized position in the pseudoknot interaction, as depicted in (B). (B) Pseudoknot depictions of xrRNABNYVV-fs, the BWYV−1 PRF pseudoknot (BWYV-PK) (adapted from Su et al. [Citation32]), the SRV-1 -1 PRF pseudoknot (SRV-1-PK) [Citation38], and xrRNAZIKV (adapted from Dilweg et al. [Citation18]). Red arrows depict the nucleotide substitutions that cause loss of XR, which were used in the−1 PRF assay. (C) Autoradiogram from−1 PRF assay for determination of frameshifting efficiencies for xrRNABNYVV-fs and xrRNAZIKV (wt), and corresponding mutant constructs (mut), with SRV-1-PK as a positive control. Image was obtained from exposure of phosphoimager screen following SDS-PAGE analysis of RRL samples containing 35S-methionine- and cysteine-labelled translation products. Frameshifting efficiencies are calculated from the ratio of − 1 PRF (FS) and non-frameshifted (NFS) products, as explained in ‘Materials and methods’, and given here as the mean (± SD) percentage from three independent experiments. Constructs of xrRNABNYVV-fs tested here correspond with those given in , and constructs of xrRNAZIKV correspond with the structure found in ZIKV isolate 15,555 (accession number MN025403) tested for XR in Dilweg et al. [Citation18].
![Figure 1. (A) Depiction of the secondary structure of xrRNABNYVV-fs, as determined in our previous study [Citation31], with some changes as described in the main text. Nucleotides are numbered from the first residue in the hp1 stem, and coloured according to the hypothesized position in the pseudoknot interaction, as depicted in (B). (B) Pseudoknot depictions of xrRNABNYVV-fs, the BWYV−1 PRF pseudoknot (BWYV-PK) (adapted from Su et al. [Citation32]), the SRV-1 -1 PRF pseudoknot (SRV-1-PK) [Citation38], and xrRNAZIKV (adapted from Dilweg et al. [Citation18]). Red arrows depict the nucleotide substitutions that cause loss of XR, which were used in the−1 PRF assay. (C) Autoradiogram from−1 PRF assay for determination of frameshifting efficiencies for xrRNABNYVV-fs and xrRNAZIKV (wt), and corresponding mutant constructs (mut), with SRV-1-PK as a positive control. Image was obtained from exposure of phosphoimager screen following SDS-PAGE analysis of RRL samples containing 35S-methionine- and cysteine-labelled translation products. Frameshifting efficiencies are calculated from the ratio of − 1 PRF (FS) and non-frameshifted (NFS) products, as explained in ‘Materials and methods’, and given here as the mean (± SD) percentage from three independent experiments. Constructs of xrRNABNYVV-fs tested here correspond with those given in Figure 4, and constructs of xrRNAZIKV correspond with the structure found in ZIKV isolate 15,555 (accession number MN025403) tested for XR in Dilweg et al. [Citation18].](/cms/asset/8751e8e5-2ee5-417a-8fa6-3d0a2746c280/krnb_a_2205224_f0001_oc.jpg)
Figure 2. Frameshifting-based XR screen leads to the discovery of a new variation of xrRNAC. (A) Schematic of pMOFS (top) that carries the split NLuc domains separated by the partially randomized frameshift cassette that is inserted during the screen (bottom). Two potential stop codons are indicated with their frame given in parentheses. Secondary structure of the xrRNAC is given as two hairpins (hp1, hp2) with numbers indicating the positions of nucleotides involved in the motif. Nucleotides that are randomized (orange in lp1, blue in spacer) are given as ‘N’. Denaturing polyacrylamide gels depict results of in vitro Xrn1 degradation assay on Hit1 and Hit2 (B) that followed as hits from the frameshifting-based XR screen, and on an SPlV5 sequence (C) that followed from subsequent GenBank searches (accession number K×883721). Boxes above the gels depict the sequences that were determined in these XR-positive hits for lp1 and spacer. Note that Hit1 differs in length due to using a different type of DNA template which omits the 5’-UGUC-3’ sequence from positions 36–39. This downstream sequence was determined to not be necessary for XR earlier [Citation31], but was added for testing XR of other frameshifting hits here in order to match the downstream sequence as present in the frameshift cassette.
![Figure 2. Frameshifting-based XR screen leads to the discovery of a new variation of xrRNAC. (A) Schematic of pMOFS (top) that carries the split NLuc domains separated by the partially randomized frameshift cassette that is inserted during the screen (bottom). Two potential stop codons are indicated with their frame given in parentheses. Secondary structure of the xrRNAC is given as two hairpins (hp1, hp2) with numbers indicating the positions of nucleotides involved in the motif. Nucleotides that are randomized (orange in lp1, blue in spacer) are given as ‘N’. Denaturing polyacrylamide gels depict results of in vitro Xrn1 degradation assay on Hit1 and Hit2 (B) that followed as hits from the frameshifting-based XR screen, and on an SPlV5 sequence (C) that followed from subsequent GenBank searches (accession number K×883721). Boxes above the gels depict the sequences that were determined in these XR-positive hits for lp1 and spacer. Note that Hit1 differs in length due to using a different type of DNA template which omits the 5’-UGUC-3’ sequence from positions 36–39. This downstream sequence was determined to not be necessary for XR earlier [Citation31], but was added for testing XR of other frameshifting hits here in order to match the downstream sequence as present in the frameshift cassette.](/cms/asset/62304178-f53b-4ddc-95fb-4bf0bb3d7dde/krnb_a_2205224_f0002_oc.jpg)
Figure 3. In vitro Xrn1 digestion assays analysing the predicted S2 element for xrRNAWCC. (A) Secondary structure of the SPlV5 xrRNA candidate sequence as present in KX883721 (top), based on the secondary structure prediction of xrRNABNYVV from KX665538 (bottom). (B) Models depicting the prediction of a pseudoknot interaction in the SPlV5 and BNYVV xrRNA motifs, showing the designation of stem elements S1 and S2, and loop elements L1 and L2. Dotted lines between S1 and L2 depict predicted tertiary interactions of adenosines with the S1 minor groove. Grey box depicts the L1-S2 pseudoknot interaction that is under consideration here. (C) Denaturing polyacrylamide gels showing results for in vitro Xrn1 digestion assays on constructs that carry the L1-S2 sequences as depicted above. Nucleotides substituted in comparison to the SPlV5 context are given in red. For PK17 and PK18 instead, nucleotides substituted in comparison to the BNYVV context are given in red.
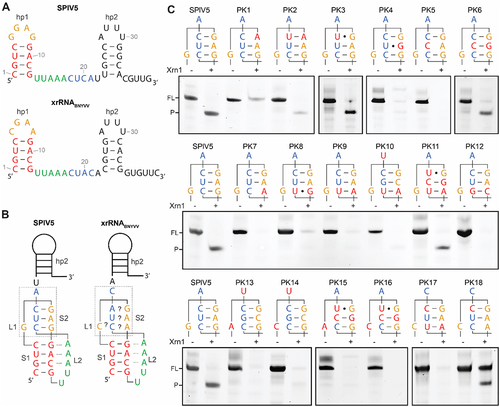
Figure 4. Determination of frameshifting efficiencies for xrRNAC, xrRNAWCC, xrRNAC with a 6-nt lp1, and corresponding constructs without a hp2. (A) Alignment showing differences between tested constructs, with dashes indicating no deviation from the frameshifting cassette as given for ‘BNYVV wt’, and empty spaces indicating deletions. Slippery sequences are shown italicized, and hp1 and hp2 stems are given in green and red, respectively. (B) Autoradiograms from−1 PRF assay that was repeated for each construct at least in triplicate. For further details, see legend to .
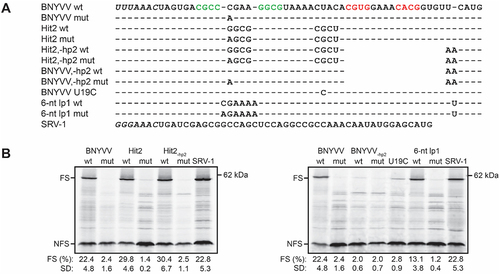
Figure 5. In vitro Xrn1 digestion assays investigating XR of known frameshifting pseudoknots. (A) Grey letters denote the leader present on RNA constructs used in this assay, followed downstream by the S1 (green) and S2 (underlined) elements, in addition to the hp2 element from xrRNABNYVV (red for the stem) for BWYV and MMTV. Sequences used were derived from accession numbers X13063 (BWYV) and D16249 (MMTV), while for IBV the ‘minimal’ pseudoknot in which its 32-nt long, functionally redundant L2 element is shortened, is used [Citation43]. (B) Denaturing polyacrylamide gels showing results for in vitro Xrn1 digestion assays on constructs as given in (A).
![Figure 5. In vitro Xrn1 digestion assays investigating XR of known frameshifting pseudoknots. (A) Grey letters denote the leader present on RNA constructs used in this assay, followed downstream by the S1 (green) and S2 (underlined) elements, in addition to the hp2 element from xrRNABNYVV (red for the stem) for BWYV and MMTV. Sequences used were derived from accession numbers X13063 (BWYV) and D16249 (MMTV), while for IBV the ‘minimal’ pseudoknot in which its 32-nt long, functionally redundant L2 element is shortened, is used [Citation43]. (B) Denaturing polyacrylamide gels showing results for in vitro Xrn1 digestion assays on constructs as given in (A).](/cms/asset/8a1bee06-de41-4c08-b545-401ec8f6ac8c/krnb_a_2205224_f0005_oc.jpg)
Supplemental Material
Download MS Word (530.7 KB)Data availability statement
The authors confirm that the data supporting the findings of this study are available within the article and its supplementary materials.