Figures & data
Figure 1. The schematic of existing circRNA formation models. Circular RNAs (circRnas) are primarily produced through back-splicing of exons (red arrow): a. intron pairing-driven circularization is guided by direct base pairing of the introns flanking complementary sequences or inverted repeats; b. RNA-binding protein (RBP)-driven circularization is produced through back-splicing of exons, which is mediated by RBPs that recognize intronic complementary sequences (ICSs) in flanking introns of circularized exons. In lariat-driven circularization, circRnas are formed during linear splicing: c. lariat-driven circularization facilitates the formation of an exon-containing lariat, which originates during an exon-skipping event (purple arrow); d. lariat-driven circularization facilitates the formation of intronic lariats, which are formed when an intron is removed during precursor mRNA (pre-mRNA) splicing (blue arrow). e. TircRNAs are formed when an intron is removed during precursor tRNA (pre-tRNA) splicing. EcircRNAs, exonic circRnas; eiciRNA, exon-intron circRnas; ciRnas, intronic circRnas; TircRNAs, tRNA intronic circRNA.
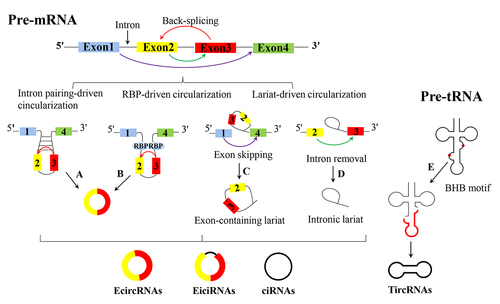
Figure 2. Schematic representation of circRnas in the pathogenesis of NAFLD. Multiple pathogenic drivers, such as insulin resistance, hepatic steatosis, oxidative stress, and inflammation, are interrelated and promote NAFLD progress to hepatic fibrosis and hepatocellular carcinoma in parallel and consistently. CircRNAs can exert diverse roles in the pathogenesis of NAFLD.
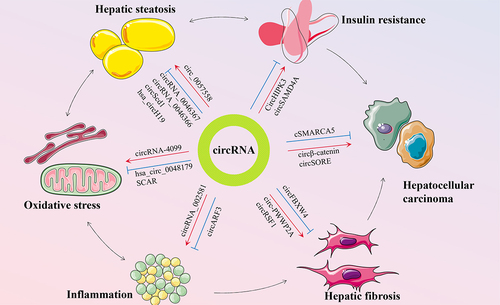
Figure 3. In vitro or in vivo experiments validation of functional mechanisms of predicted circRNAs. NAFLD, non-alcoholic fatty liver disease; CPEB1, cytoplasmic polyadenylation element-binding protein 1; PTEN, phosphatase and tensin homolog; AMPK, AMP-activated protein kinase; mTOR, mammalian target of rapamycin; PPARα, peroxisome proliferator-activated receptor α; CPT2, carnitine palmitoyltransferase 2; ACBD3, acyl-CoA binding domain containing 3; CPT1, carnitine palmitoyl transferase 1; ABCA1, ATP-binding cassette transporter A1; ROCK1, rho-kinase 1; AMPK, AMP-activated kinase; JAK2, Janus kinase 2; STAT5, signal transducer and activator of transcription 5; GPX4, glutathione peroxidase 4; CypD, cyclophilin D; mPTP, mitochondrial permeability transition pore; mROS, mitochondrial reactive oxygen species.
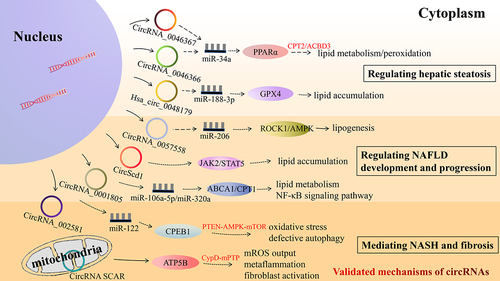
Figure 4. Possible sorting mechanisms and functional pathways for exosomal ncRnas. ncRnas are sorted into exosomes via two potential routes: (i) the RNA motif and RBP-mediated pathway: ncRnas with specific ex-motifs are recognized by some RBPs and packaged into exosomes via the formation of an ncRNA-RBP binary, or ternary, complex. (ii) the ncRNA-related pathway: changes in miRNA levels cause circRNA to be packed into exosomes and vice versa. After entering a recipient cell, exosomal ncRnas (i) are separated and released to perform functions specific to each ncRNA or (ii) remain in the circRnas-miRNA or exosomal-sorting-RBPs binding complex without participating in any biological function. RBPs, RNA-binding proteins; ex-ncRnas, exosomal ncRNA; MVBs, multivesicular bodies; AGO, argonaute; ESCRT, endosomal sorting complex required for transport; miRisc, miRNA-induced silencing complex.
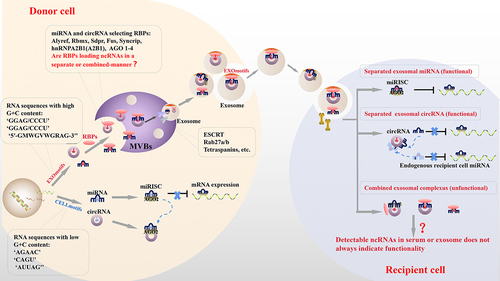
Figure 5. Strategies used to target circRnas. a siRNa/shRNA targeting the back-splice junction of circRnas induces circRnas cleavage. b CircRNAs expression plasmid leads to circRnas overexpression. C gold nanoparticle-mediated delivery of circRnas. d exosome-mediated delivery of circRnas. e antisense oligonucleotides (ASO) bind to targeted circRnas through complementary pairing to induce circRnas cleavage. f cre-lox system-based conditional circRNA knockdown to induce circRNA cleavage. g CRISPR/Cas9 disrupting the flanking intronic complementary sequence induces circRNA cleavage. h CRISPR/Cas13 directly targeting the back-splice junction of circRnas induces circRNA cleavage.
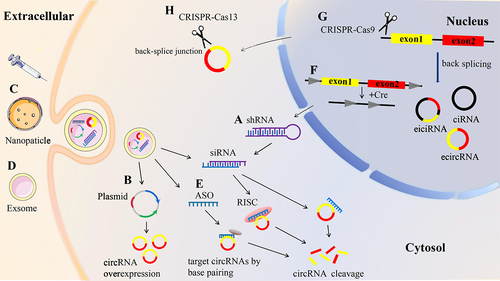