Figures & data
Figure 1. The chaperone-assisted selective autophagy (CASA) complex. (A) Schematic representation of the CASA complex. BAG3 (green) mediates the assembly of the CASA complex, thereby linking chaperones of the HSPA family (purple) to small heat shock proteins such as HSPB8 (blue). The HSPA-associated E3 ubiquitin ligase STUB1 (orange) interacts with the complex for the ubiquitination of the bound client protein. (B) Engulfment of the CASA complex by LC3-decorated phagophore membrane is facilitated by the autophagic cargo receptor SQSTM1, leading to formation of double-membrane vesicles (called autophagosomes) and final degradation of its content by fusion with the lysosomes.
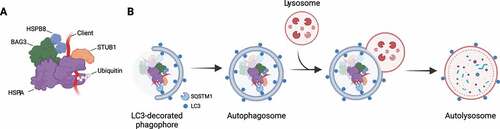
Figure 2. CASA members with their protein domains and corresponding interactors. Schematic representation of the protein domains of HSPA, BAG3, HSPB8 and STUB1 and related interactors.
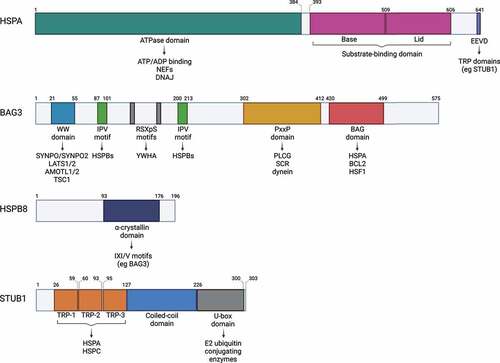
Figure 3. Steps of the HSPA substrate binding/release cycle. (1) DNAJ (orange) mediates the delivery of nascent or misfolded proteins to ATP-bound HSPA (purple). (2) Hydrolysis of ATP to ADP results in a conformational change of HSPA: the “lid” of the substrate-binding domain closes to tightly bind the substrate. DNAJ dissociates from the chaperone complex. (3) A nucleotide exchange factor (NEF, pink) binds to HSPA, facilitating the exchange of ADP for ATP. (4) ATP binding to the ATPase domain induces the opening of the “lid”, thereby enabling substrate release from HSPA.
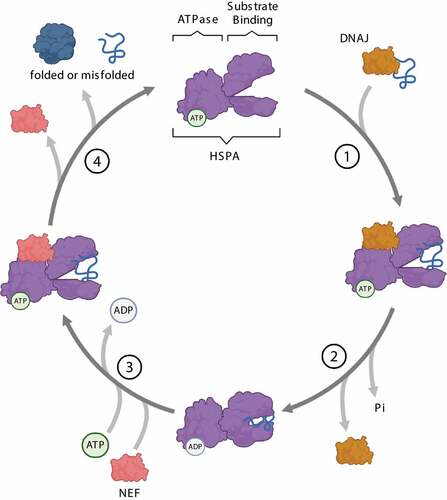
Figure 4. Mechanisms of CASA dynamics and activities. In unstressed conditions, damaged and misfolded substrates are routed to the UPS by HSPA-STUB1-BAG1 complex. Upon proteotoxic stress, HSPA preferentially interacts with STUB1-BAG3 and HSPB8, forming the CASA complex. The CASA complex utilizes the dynein machinery to route substrates to the MTOC, where they are deposited at the aggresome. Alternatively, HSPB8 drives ubiquitinated substrates and SQSTM1 microaggregates formation and routing to the aggresomes. Aggresomes are subsequently processed by the ALP. CASA complex is also involved in granulostasis upon stress, by promoting stress granule disassembly through the extraction of misfolded substrates (and DRiPs) from the stress granule and their routing to ALP degradation.
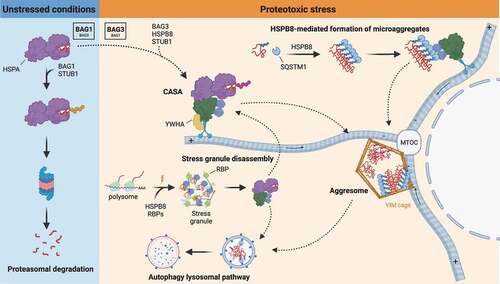
Figure 5. CASA complex in stress signaling pathways. The CASA complex directly regulates signaling pathways involved in the adaptive response to stress. (A) EIF2AK1/HRI-EIF2A signaling axis is activated by pathogens or proteotoxicity. In response to pathogens, HSPB8 is recruited to signalosomes and releases EIF2AK1, which promotes EIF2A phosphorylation. Alternatively, in response to proteotoxic stress, the binding of ubiquitinated substrates to HSPA-BAG3 activates EIF2AK1 signaling. Phosphorylated EIF2A stimulates transcription factors (e.g., ATF4) of the integrated stress response (ISR). (B) Hippo pathway and YAP1-WWTR1 signaling is activated by mechanical stress or proteotoxicity. Upon mechanical stress, BAG3 binds LATS1 or LATS2 and AMOTL1 or AMOTL2, thereby suppressing the cytoplasmic sequestration or degradation of YAP1-WWTR1, allowing their translocation into the nucleus. Alternatively, upon proteotoxic stress, ubiquitinated substrates compete with AMOTL2 for LATS1 binding. AMOLT2 displacement suppresses YAP1 phosphorylation and permits BAG3-mediated YAP1 translocation into the nucleus and expression of its target genes. (C) NFE2L2-KEAP1 signaling axis. In physiological conditions, KEAP1 is bound to NFE2L2 and favors its CUL3-mediated ubiquitination and proteasomal degradation. Upon proteasome inhibition, HSPB8 favors the sequestration of ubiquitinated substrates into SQSTM1 oligomers. Phosphorylated SQSTM1 bodies recruit KEAP1, freeing NFE2L2. Unbound NFE2L2 translocates into the nucleus where it promotes the transcription of stress-responsive genes.
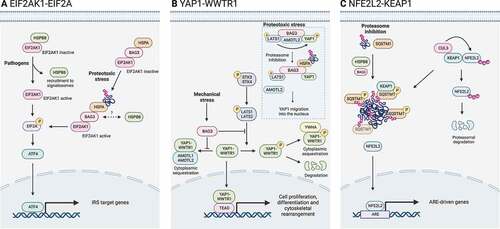
Figure 6. CASA-mediated degradation of Z-disk component FLNC. Mechanically unfolded and damaged forms of FLN are recognized and degraded by the CASA pathway. Here, BAG3 closely cooperates with HSPB8 (the “holdase”) and regulates the ATP-dependent chaperone cycle of HSPA, necessary for substrate processing. STUB1-mediated ubiquitination and subsequent recruitment of the autophagic receptor SQSTM1 initiates autophagosome formation, followed by lysosomal fusion for degradation.
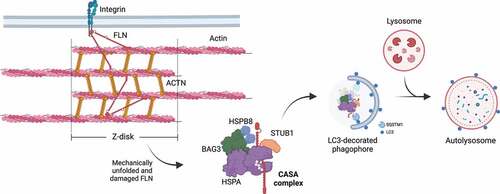
Table 1. Overview of reported disease-causing mutations in BAG3, HSPB8 and STUB1.