Figures & data
Figure 1. Structural and functional components of KDM5A: a) schematic showing various domains of KDM5A: (I) catalytic domain of KDM5A is split into two parts – Jumonji N (JmJN) and Jumonji C domains (JmJC). While JmJC is primarily responsible for catalytic activity of the molecule, JmJN provides the structural integrity to catalytic cassette. (II) ARID domain is the DNA binding domain and recognizes GC rich sequences. (III) PHD fingers- KDM5A has three plant homeodomains. PHD1 along with ARID domain are located between JmJN and JmJC. Along with the zinc finger, these five components form the catalytic cassette of the molecule. PHD1 also shows preferential binding for unmethylated H3K4 residues. While binding preference of PHD2 is not known, PHD3 has been shown to specifically binds H3K4me3 residues. (IV) KDM5 family contain a conserved PLU-1 like domain whose functionality is not known currently. (V) between PHD2 and PHD3 are two domains – LxCxE motif responsible for protein’s interaction with pocket protein family and PID (PAR interacting domain) which is important for PAR recognition and interaction. c) AlphaFold predicted structure of catalytic cassette of KDM5A showing spatial arrangement of different domains is shown: different domains are highlighted in different colours as indicated. Critical residues for different domains that are highlighted – Jumonji C [HIS483, GLU485], ARID [LYS152] and PHD1 [TRP335]. c) AlphaFold predicted structure and spatial arrangement of domain involved in KDM5A catalytic cassette without any linker chains is shown.
![Figure 1. Structural and functional components of KDM5A: a) schematic showing various domains of KDM5A: (I) catalytic domain of KDM5A is split into two parts – Jumonji N (JmJN) and Jumonji C domains (JmJC). While JmJC is primarily responsible for catalytic activity of the molecule, JmJN provides the structural integrity to catalytic cassette. (II) ARID domain is the DNA binding domain and recognizes GC rich sequences. (III) PHD fingers- KDM5A has three plant homeodomains. PHD1 along with ARID domain are located between JmJN and JmJC. Along with the zinc finger, these five components form the catalytic cassette of the molecule. PHD1 also shows preferential binding for unmethylated H3K4 residues. While binding preference of PHD2 is not known, PHD3 has been shown to specifically binds H3K4me3 residues. (IV) KDM5 family contain a conserved PLU-1 like domain whose functionality is not known currently. (V) between PHD2 and PHD3 are two domains – LxCxE motif responsible for protein’s interaction with pocket protein family and PID (PAR interacting domain) which is important for PAR recognition and interaction. c) AlphaFold predicted structure of catalytic cassette of KDM5A showing spatial arrangement of different domains is shown: different domains are highlighted in different colours as indicated. Critical residues for different domains that are highlighted – Jumonji C [HIS483, GLU485], ARID [LYS152] and PHD1 [TRP335]. c) AlphaFold predicted structure and spatial arrangement of domain involved in KDM5A catalytic cassette without any linker chains is shown.](/cms/asset/9be641f9-305f-4a15-9edb-61e12a6c8b37/kepi_a_2268813_f0001_oc.jpg)
Figure 2. Pocket protein interaction site of KDM5A: KDM5A has a T/E1A binding domain located between PHD2 and PHD3. It contains LxCxE motif which is able to interact with all three pocket proteins pRb, p107 and p130. This interaction can be abrogated by changing a single residue (LxCxE to LxCxK). Beside the LxCxE motif, KDM5A also has a non-T/E1A binding domain through which it can interact with pRb protein. Amino acid residues indicating the position of relevant domains/motifs are shown.
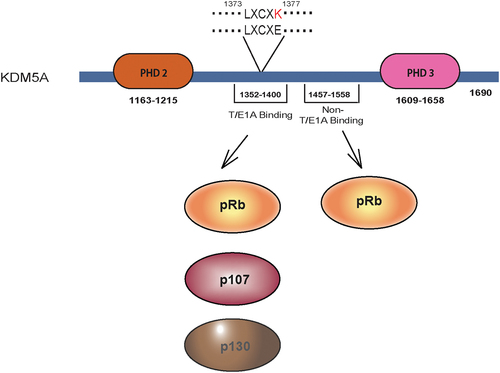
Figure 3. Various roles of KDM5A in different type of cancers: KDM5A is overexpressed in many types of cancer where it drives cancer proliferation, invasion and drug resistance. Some of these interaction as shown above are as follows - (I) in acute myeloid leukaemia – NUP98 protein and PHD3 of KDM5A forms a fusion protein which regulate the expression of HOX a gene cluster. This fusion protein alters the expression level of HOX a cluster leading to erroneous differentiation, (II) in ovarian cancer – KDM5A overexpression promotes proliferation and epithelial-to-mesenchymal transition in SKOV3 cells. It was also shown to be playing in PTX resistance in SKOV3 cells, (III) in prostate cancer – KDM5A localizes on tumour suppressor and differentiation genes such as KLF-4 and E-cadherin to reduce their expression. Reduction in these gene correlate with higher malignancy of cancer, (IV) in breast cancer – overexpression of KDM5A drives the drug resistance by downregulating p16 and bak-1. It also helps in cancer proliferation by regulating p27 along with p16, (V) in lung cancer – KDM5A reduces the expression of p16, cyclin D1 etc. To drive cancer proliferation, invasion and drug resistance. In small cell lung cancer, KDM5A causes carcinogenesis by downregulates Notch1 and Notch2, (VI) in gastric cancer – KDM5A promotes the tumour activity by downregulating the cyclin dependent kinase inhibitors (CDKI: p16, p21 & p27). Transactivation of vascular endothelial growth factor (VEGF) by KDM5A also plays important role in gastric tumorigenesis.
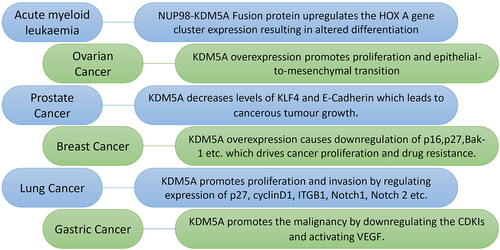
Figure 4. Models showing various complexes of pocket proteins and KDM5A that are involved in differentiation, proliferation and senescence. (I) during proliferation E2F4 forms a complex with KDM5A with the help of p130. This interaction is limited to early G1 and the model proposes various members such as sin3 HDAC, dREAM complex components and SWI/SNF are a part of this complex to repress transcription at E2F-responsive promoter. (II) during differentiation, KDM5A is part of following complexes; (i) KDM5A interacts with pRb directly to regulate gene activity of BRD2 and BRD8. pRb sequesters KDM5A, preventing the H3K4me3 removal, which leads to increased transcriptional activity at BRD2 and BRD8 promoters. (ii) similar to the complex in proliferating cells, KDM5A interacts with E2F4 indirectly with the help of p130 along with various components like dREAM to form a repressor complex during differentiation. (III) during senescence, pRb, sin3HDAC and KDM5A forms a complex at the E2F responsive promoter, most likely with an E2F transcription factor, to repress transcription.
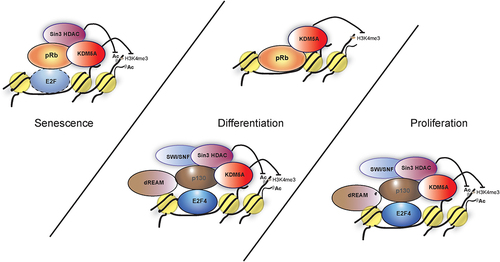
Figure 5. Various protein–protein interactions of KDM5A for its chromatin recruitment: KDM5A is recruited by its many partners to the chromatin to regulate the transcription in a demethylase dependent/independent manner. Some of these interactions, as shown above, are as follows: (I) cell cycle – KDM5A interacts with pocket protein family member – p130 and is recruited to E2F4 responsive promoter in early G1 for repression of target genes, (II) notch signalling – KDM5A is recruited by RBP-J to various notch target gene to regulate their transcription, (III) IFN signalling – Piasy interacts with JmJC domain of KDM5A and recruits it to IFNI promoter. KDM5A represses the IFNI gene activity by removing H3K4me3 marks, (IV) differentiation – during development, KDM5A interacts with chromatin remodellers such as sin3B and NuRD. KDM5A, along with NuRD, controls developmentally regulated genes, (V) DNA damage repair – upon DNA damage, PARP1 generates PAR chains at the damage sites which helps in recruitment of KDM5A. KDM5A carries out the transcriptional repression, (VI) gene activity – KDM5A is also shown to interact with MAD1 transcription factor to regulate the gene activity by controlling H3K4me3 levels, (VII) circadian clock maintenance – KDM5A works in a demethylase independent manner and prevents the interaction of HDAC1 with CLOCK-BMAL1. Accumulation of H3K4me3 and H3K9ac leads to higher gene expression. (please see the text for details).
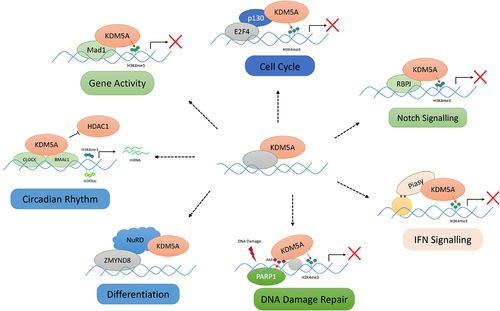
Data availability statement
Data sharing is not applicable to this article as no new data were created or analysed in this study.