Figures & data
Figure 1. A-to-I RNA editing and the evolution of adar S > G auto-recoding site in insects. (a) A-to-I RNA editing mediated by ADAR protein. I is recognized as G by cellular machineries. (b) Predictions made by the adaptive RNA editing hypothesis. A and G alleles are fitter under different conditions while RNA editing could adjust the relative proportions of two alleles. When a is fitter under a particular condition, the editing level decreases to represent more A, and vice versa. (c) The adar S > G auto-recoding site forms a negative feedback loop that stabilizes the RNA editing efficiency. (d) Evolution of adar S > G site in insects. Editable serine codons are colored in red; uneditable serine codons are colored in blue; glycine codons are colored in orange. The phylogenetic tree of all diptera species was colored with the codon classification of adar S > G site.
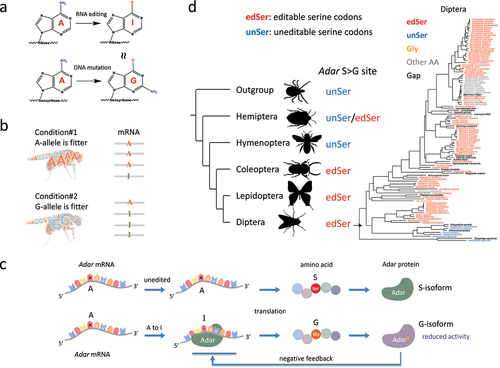
Figure 2. Putative evidence for adaptive RNA editing based on comparative genomic analysis. (a) For a potentially adaptive A-to-I RNA editing site, the editable status is fitter than hardwired G. (b) The genomic A-to-G mutations should be depleted at adaptive RNA editing sites because the hardwired G-allele would abolish the flexibility conferred by RNA editing. (c) As a control, unedited adenosines do not have a clear (predictable) fitness change after A-to-G mutation. (d) For the unedited adenosines in D. melanogaster. The genomic replacement of A-to-G should be frequently observed in the phylogeny.
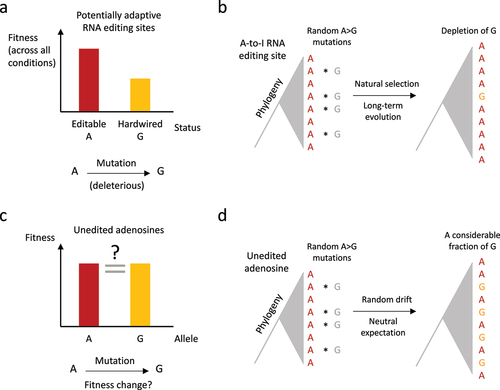
Figure 3. The basic concepts used for downstream analyses. (a) In 113 diptera species, the codons and AAs corresponding to the adar S > G site were displayed. (b) Classification of unedited adenosines in D. melanogaster adar gene. (c) Definition of original codon/AA and derived codon/AA. Transition rates were calculated using the original and derived codons/AAs.
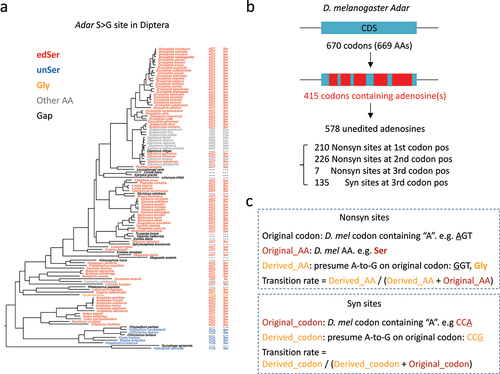
Figure 4. A-to-G transition in other diptera species is significantly avoided for the RNA editing site in drosophila Adar. (a) Mean ± S.E. (standard error) of the expected transition rate from original AA (codon) to derived AA (codon). Unedited adenosines were used and shown as squares and whiskers. The observed transition rate at adar S > G site was labeled in the plot. (b) Definition of gapped diptera species and histogram showing the numbers of gaps at each site. (c) Mean ± S.E. (standard error) of the expected transition rate using unedited adenosines with > 18 gapped species in diptera.
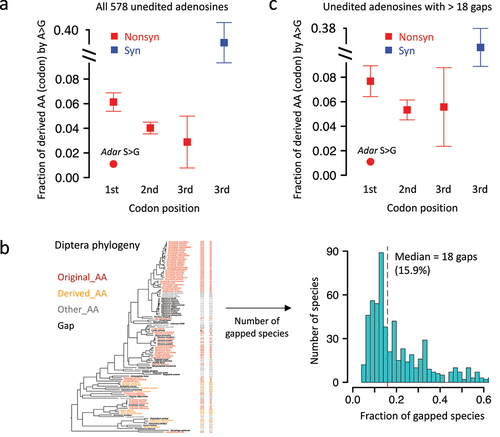
Figure 5. Examples of unedited nonsynonymous adenosines in adar CDS with high transition rate. The information of each site (CDS position, AA position, AA change of the site and the numbers of each category) was displayed in the plot.
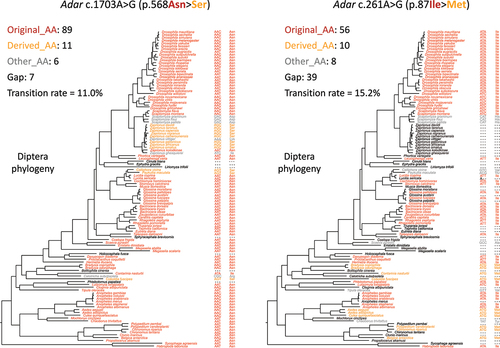
Figure 6. Editing levels under different conditions. (a) S > G auto-recoding level in three different drosophila species under 25°C and 30h. (b) Global editing level of shared editing sites across three species. (c) and (d) Display two examples of elevated editing level under high temperature. The trend is conserved across three species. D. mel, D. melanogaster; D. sim, D. simulans; D. pse, D. pseudoobscura. (e) S>G auto-recoding level in 10 samples of different developmental stages and head/body of male/female adults. Data were retrieved from our previous study [Citation8].
![Figure 6. Editing levels under different conditions. (a) S > G auto-recoding level in three different drosophila species under 25°C and 30h. (b) Global editing level of shared editing sites across three species. (c) and (d) Display two examples of elevated editing level under high temperature. The trend is conserved across three species. D. mel, D. melanogaster; D. sim, D. simulans; D. pse, D. pseudoobscura. (e) S>G auto-recoding level in 10 samples of different developmental stages and head/body of male/female adults. Data were retrieved from our previous study [Citation8].](/cms/asset/c1416aff-254b-4d29-9fa7-fcb1c83cc810/kepi_a_2333665_f0006_oc.jpg)
Figure 7. A-to-G transition in other diptera species is significantly avoided for the Ile>Met (I > m) recoding site in Syt1. (a) In 113 diptera species, the codons and AAs corresponding to the Syt1 I > M site were displayed. (b) Mean ± S.E. (standard error) of the expected transition rate from original AA (codon) to derived AA (codon). Unedited adenosines were used and shown as squares and whiskers. The observed transition rate at Syt1 I > M site (rate = 0) was labeled in the plot. (c) Mean ± S.E. of the expected transition rate using unedited adenosines with > 17 (median value for all sites) gapped species in diptera.
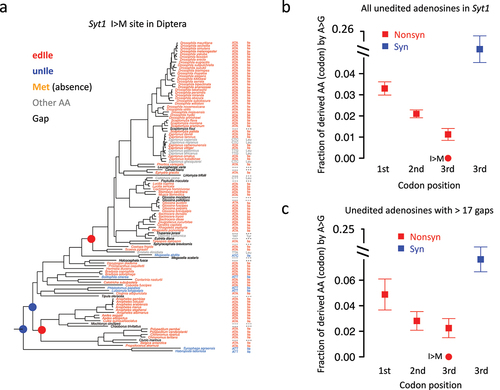
Figure 8. Checking the editing status of adar S > G site in representative diptera species. Editing event at this position was already observed in the drosophila genus. For non-drosophila clades, head transcriptomes of four species were downloaded and mapped to the adar sequence. Accession IDs of the transcriptome data were given above each sample. Screenshots of IGV visualization at S > G site was displayed. Not all RNA-Seq reads were displayed. The detected editing event and the editing level were highlighted.
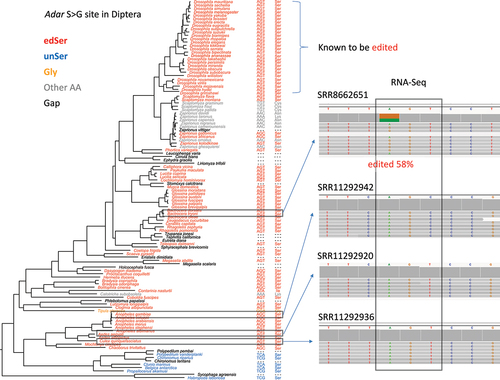
Data availability statement
The analysis of the Adar auto-recoding codon involves the reference sequences of 113 Diptera species which were downloaded from NCBI https://www.ncbi.nlm.nih.gov/. The detailed links of the data were supplemented in our previous works [Citation27,Citation28]. The phylogeny of Diptera was also retrieved from our previous study [Citation27]. The head transcriptomes of four Diptera species were downloaded from NCBI with the following accession IDs: Anopheles gambiae (SRR11292942), Aedes aegypti (SRR11292920), Culex quinquefasciatus (SRR11292936), and Bactrocera tryoni (SRR8662651). The brain transcriptomes of three Drosophila species D. melanogaster, D. simulans, and D. pseudoobscura were downloaded from NCBI with accession number SRP074828. The developmental transcriptomes and RNA editomes of D. melanogaster were retrieved from our previous study [Citation8]