Figures & data
Figure 1. A working model for photosynthesis-respiration interactions in tobacco under different growth conditions. The “ATP/NADPH imbalance” in the chloroplast refers to the shortage in production rate of ATP relative to NADPH, assuming that only linear electron transport is supplying these to the Calvin-Benson cycle. The Calvin-Benson cycle demand for ATP relative to NADPH decreases as the ratio of carboxylation to oxygenation by ribulose bisphosphate carboxylase oxygenase increases. Compared to normal growth conditions, this demand is low during growth at elevated atmospheric concentrations of CO2 and high during growth under water deficit. One means to correct the ATP/NADPH imbalance is to export NADPH to the cytosol via a metabolite shuttle such as the malate valve. Hence, to correct the chloroplast ATP/NADPH imbalance, and relative to normal growth conditions, growth at elevated CO2 may reduce NADPH export to the cytosol while water deficit may increase NADPH export to the cytosol. Compared to normal growth conditions, growth at elevated CO2 increases the Calvin-Benson cycle supply of triose phosphates to the cytosol, while water deficit decreases this supply. The mitochondrial electron transport chain oxidizes NAD(P)H that is being supplied either directly from the chloroplast via the malate valve, or indirectly via the metabolism of chloroplast-derived triose phosphates by cytosolic glycolysis and the mitochondrial tricarboxylic acid cycle. Under normal growth conditions, it is likely that most electrons are consumed by cytochrome oxidase, allowing the mitochondrion to supply sufficient ATP to the cytosol for sucrose synthesis. Under water deficit, cytochrome oxidase protein amount declines while alternative oxidase protein amount increases; and alternative oxidase becomes a necessary electron sink in the photosynthetic cell. This reduces the mitochondrial supply of ATP to the cytosol, but ATP demand for sucrose synthesis is likely low regardless, due to the relatively low rates of chloroplast triose phosphate production. Growth at elevated CO2 increases both cytochrome oxidase and alternative oxidase protein amounts, but little information is available regarding the partitioning of electrons between the two pathways under these conditions. Nonetheless, AOX does become a necessary electron sink, in this case to prevent a buildup of excess carbohydrate in the photosynthetic cell due to the relatively high rates of chloroplast triose phosphate production. See text for additional details. AOX, alternative oxidase; COX, cytochrome oxidase; TP, triose phosphate.
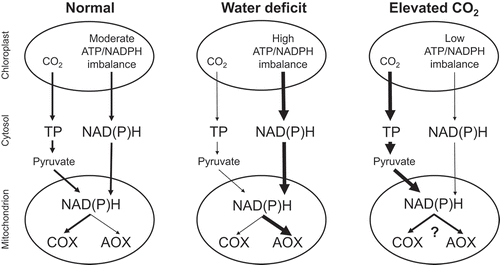
Figure 2. Chloroplast ATP synthase is a master regulator of photosynthesis. Light-driven electron transport generates NADPH and a proton gradient between the stroma and lumen. The proton gradient provides the proton motive force for energy (ATP) generation, but the lumen protons can also engage feedback biochemical controls that slow electron transport. The activity of the chloroplast ATP synthase will determine the extent to which the proton gradient is either dissipated to provide ATP for the Calvin-Benson cycle, or allowed to build up and hence engage the feedback controls on electron transport. Stromal Pi and ADP are the substrates for ATP synthase. Stromal Pi has also been hypothesized to act as an allosteric effector of ATP synthase activity (see text) and/or as a signal molecule able to influence ATP synthase protein amount (this work). NPQ, non-photochemical quenching; pmf, proton motive force.
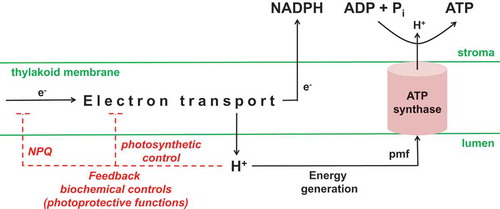
Table 1. Predicted relative concentrations of stromal inorganic phosphate (Pi) correlate with measured changes in chloroplast ATP synthase (AtpB subunit) and Rubisco (RbcS subunit) protein amounts in different growth environments (water deficit or elevated atmospheric concentrations of CO2) and across plant lines with altered respiration (alternative oxidase knockdowns and overexpressors). See text for further details. Based on data from references 25–28.