Figures & data
Figure 1. The Eulerian thermohaline stream function, computed as described by Döös et al. (Citation2012). The contour interval is 4 Sv (), where red represents clockwise and blue anticlockwise. The most common water masses found in the Atlantic Ocean are marked with their abbreviations at their TS-space characteristics (Talley, Citation2011). SACW, NACW = South and North Atlantic central waters, MW = Mediterranean water, AAIW = Antarctic intermediate water, NADW = North Atlantic deep water and AABW = Antarctic bottom water. The marked area is suggested to represent the circulation of the Atlantic Ocean in the thermohaline stream function.
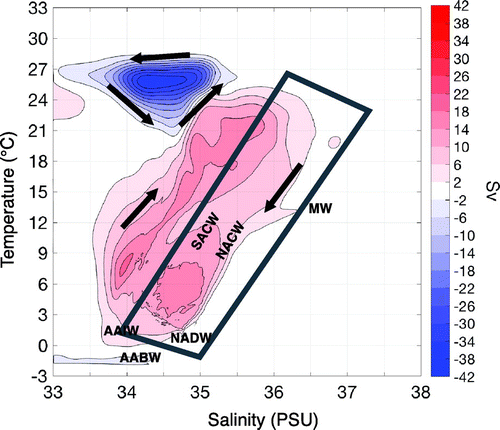
Figure 2. The region of the Atlantic Ocean used in the present study. The grey lines show the southern (17S) and northern (58
N) boundaries delimiting the domain. The blue lines represent the path of water starting at the southern boundary and continuing either back to the southern boundary or to the northern boundary. The red lines represent the path of water starting at the northern boundary, which can go either back to the northern boundary or southwards and exiting the domain at the southern boundary. The grey dashed line is the equator.
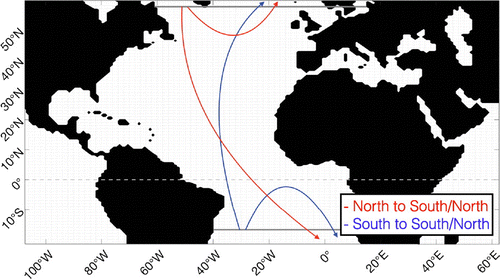
Figure 3. Discretization of the Lagrangian thermohaline stream function. (a) shows a trajectory with transport crossing a grid cell, passing the walls (
, j, k) and (i, j, k). U and V are the transports in zonal and meridional directions located at the wall the trajectory crosses.
is the temperature in the middle of the grid box.
and
are the lengths of the grid box. (b) shows the same trajectory crossing the same grid box as in a, but with superimposed isotherms (red) and isohalines (blue) that lie inside the grid box. The isotherms and isohalines have the indices m and l, respectively. c) shows the same trajectory in TS-space, crossing the same isotherms and isohalines as in panel b, but now the isotherms and isohalines represent a new coordinate system.
and
are the distances between isotherms and isohalines.
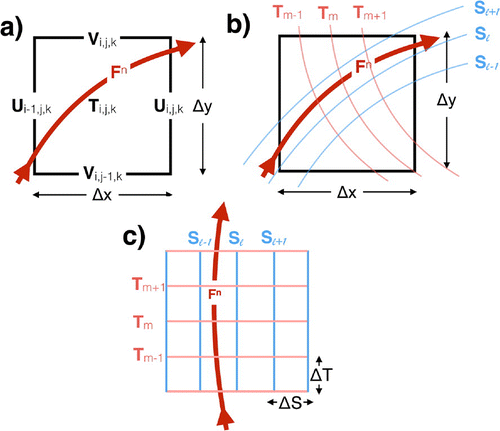
Figure 4. The Atlantic meridional overturning stream function according to (a) the Lagrangian computation with TRACMASS and (b) the Eulerian computation using the first 10 years of data. (c) shows the difference between the Lagrangian and Eulerian stream functions. Panels a, b and c have the same colourbar, with 2 Sv as contour intervals.
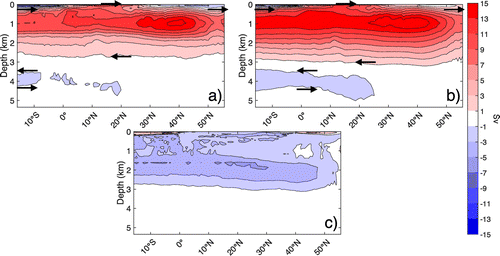
Figure 5. Six trajectories going from north to north (blue) and six trajectories going from south to north (red) illustrated in (a) latitude–longitude space and (b) temperature–salinity space.
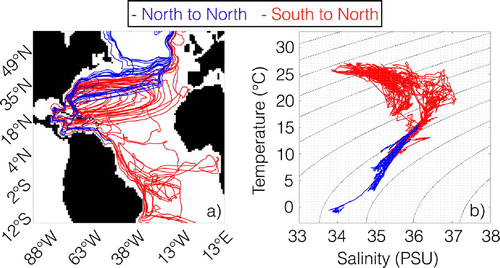
Figure 6. The northward transport in the Atlantic Ocean computed with the TRACMASS scheme displayed as (a) the Lagrangian meridional overturning stream function, (b) the Lagrangian barotropic stream function, (c) the Lagrangian latitude–density stream function and (d) the Lagrangian latitude–temperature stream function. The contour interval for all stream functions displayed is set to 2 Sv.
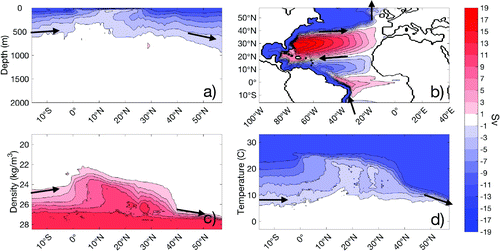
Figure 7. (a) The Lagrangian thermohaline stream function computed for the northward transport in the Atlantic Ocean. The contour interval is set to 2 Sv (). The initial positions of trajectories in TS-space are shown as green dots, whereas their end positions in TS-space are shown as purple dots. Superimposed are isopycnals for
(solid) and
(dashed), both with intervals of 1. (b) shows the same Lagrangian thermohaline stream function as in (a), but with the global Eulerian thermohaline stream function (cf. Fig. ) superimposed with contours. Both stream functions have the same colourbar, with a contour interval of 2 Sv.
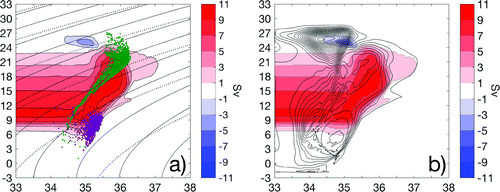
Figure 8. The Lagrangian divergence of temperature and salinity computed from trajectories simulated with the TRACMASS scheme. (a) shows the Lagrangian divergence of temperature for the northward transport in the Atlantic Ocean, displayed as MW, whereas (b) shows the Lagrangian divergence of salinity for the northward transport in the Atlantic Ocean, as PSU kg
. (c) shows the Lagrangian divergence of temperature for the water mass starting in north, travelling back north in MW
, whereas (d) shows the Lagrangian divergence of salinity for the same water mass in PSU kg
.
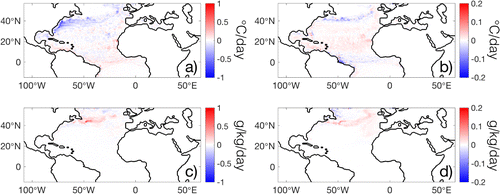
Figure 9. One specific trajectory that illustrates the conversion of temperature and salinity in the Atlantic Ocean driven by a mixing rather than air–sea interactions. The trajectory is red if it is below the mixed layer depth, whereas it is blue if it is inside the mixed layer. The mixed layer depth chosen is the deepest mixed layer for all years of data (1850–2005). (a) shows the trajectory in longitude–latitude space, (b) in latitude–depth space, (c) in salinity–temperature space, superimposed on the Lagrangian thermohaline stream function with 2 Sv as contour lines, (d) in latitude–temperature space, and (e) latitude–salinity space.
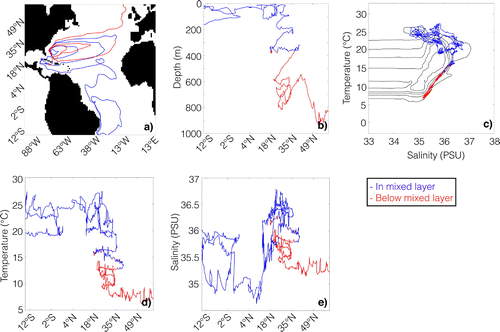
Figure A1. Illustration of a trajectory that passes through a grid box crossing the walls (, j, k) and (i, j, k).
is the temperature flux of the trajectory at grid wall i, j, k, whereas
is the temperature flux of the trajectory at
, j, k.
,
and
are the dimensions of the grid box.
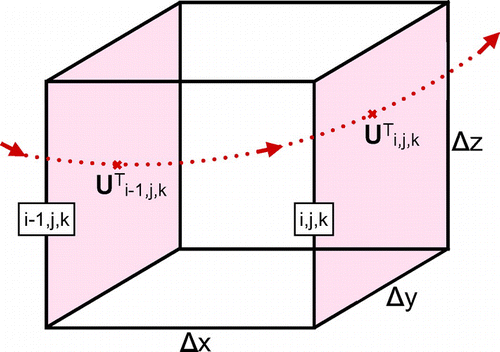