Figures & data
Figure 2. Thermal boundary conditions considered for the block 1 in multiblock decomposition (cross-sectional view).
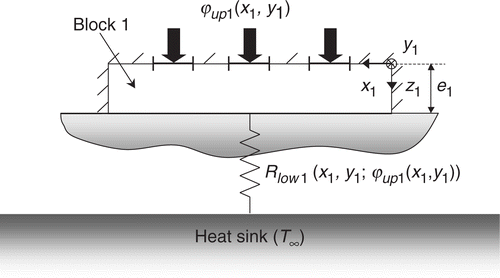
Figure 3. Thermal boundary conditions considered for the block 2 in multi-block decomposition (cross-sectional view).
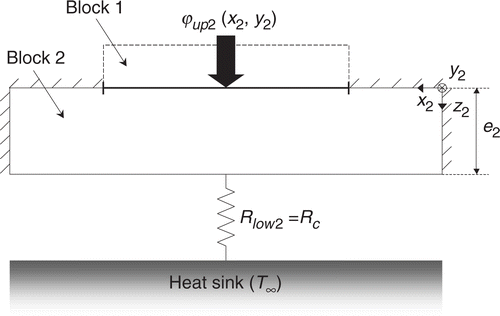
Figure 4. Principle of the iterative process for solving the 3D steady-state heat conduction direct problem.
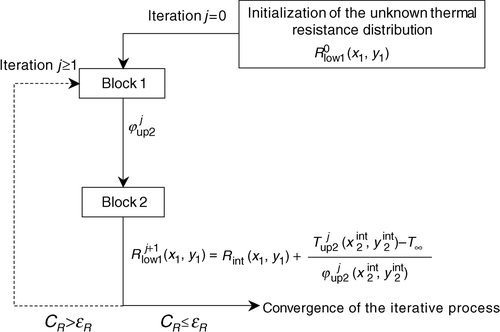
Figure 5. Heat source configuration on the upper face of the block 1 for the validation of the direct problem.
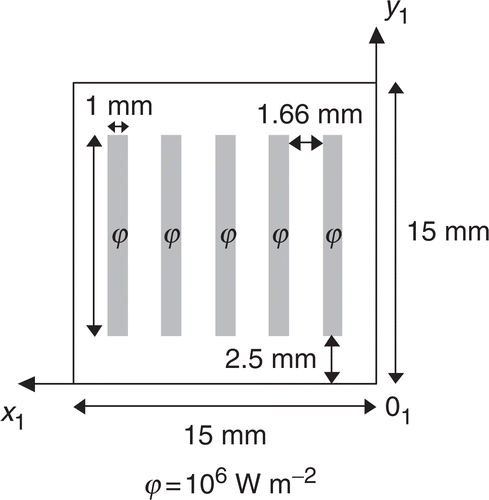
Figure 6. Interface thermal resistance distribution (K m2 W−1): Rint(x1,y1) = 0.5 × sin(x1.y1) − validation of the direct problem.
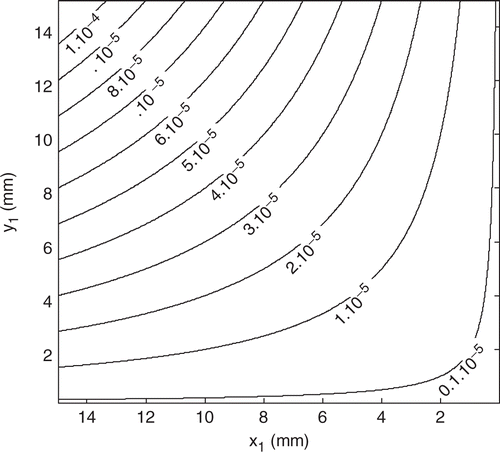
Table 1. Thermal properties and dimensions of the microelectronic device for the validation of the direct problem
Figure 7. Validation of the direct problem–temperature profiles on the upper face of the block 1: (a) for y1 = 7.5 mm, (b) for x1 = 7.5 mm.
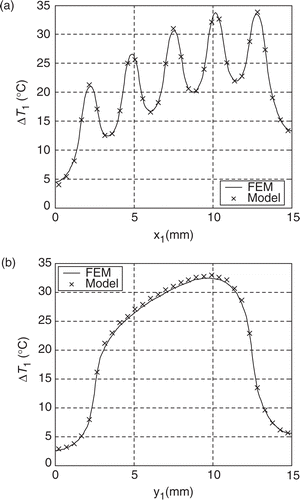
Figure 8. Examples of parametrization of the interface thermal resistance distribution: (a) 4-zone partition, (b) 25-zone partition, (c) 64-zone partition.
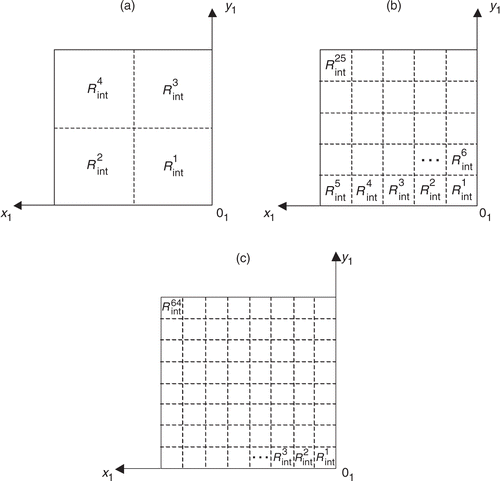
Figure 9. Exact interface thermal resistance distributions (Km2 W−1) investigated in the numerical experiments.
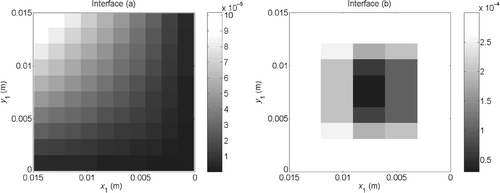
Table 2. Spatial characteristics of the exact interface thermal resistance distributions
Figure 10. Heat source configurations on the upper face of the block 1 investigated in the numerical experiments.
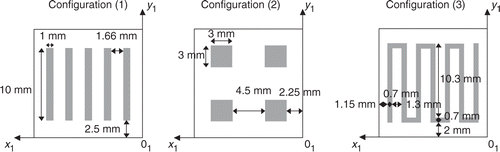
Figure 11. Sensitivity coefficients distribution (Wm−2) of η(β) to the component n°33 of β computed for the HSC (1) and .
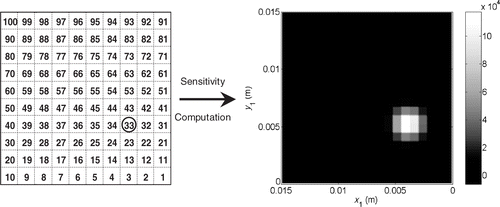
Figure 13. Evolution of the estimated distributions (Km2 W−1) (interface (a), HSC (1), and σ = 0): (a) 5 iterations, (b) 20 iterations, (c) 50 iterations, (d) 100 iterations.
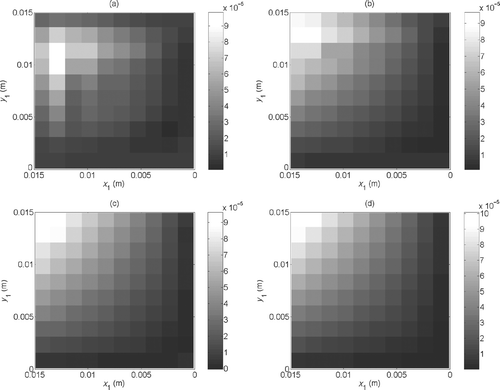
Figure 14. Evolution of the functional J for the estimation of the interface (a) with the three HSC, and σ = 0.1 K.
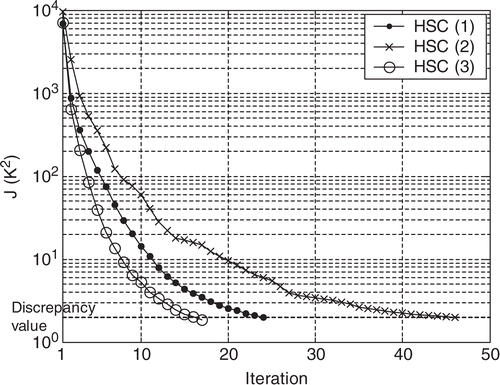
Figure 15. Evolution of the functional J for the estimation of the interface (b) with the three HSC, and σ = 0.1 K.
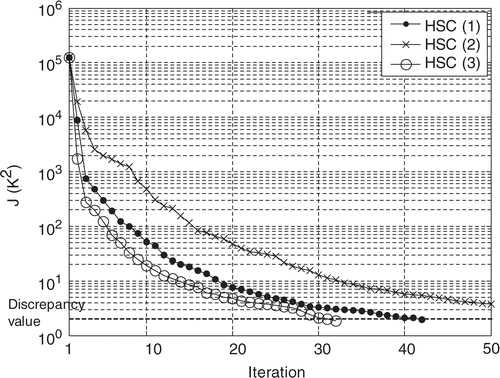
Figure 18. Relative error due to measurement errors for the estimation of the interface (a) with the HSC (3) and σ = 0.1 K.
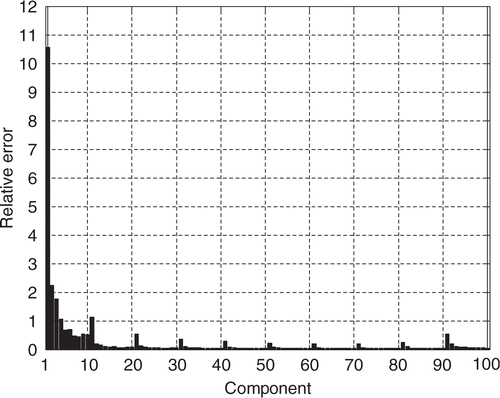
Figure 19. Relative error due to measurement errors for the estimation of the interface (b) with the HSC (3) and σ = 0.1 K.
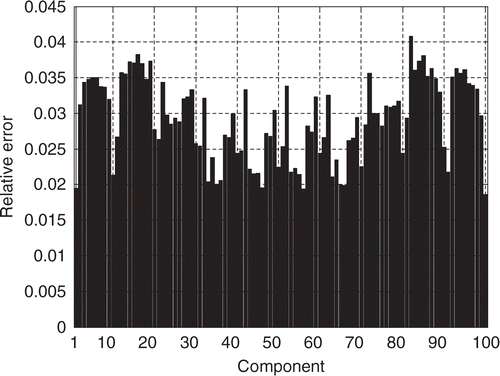
Figure 20. Evolution of the functional J for the estimation of the interface (a) with the HSC (1), σ = 0.1 K, and different update parameters K.
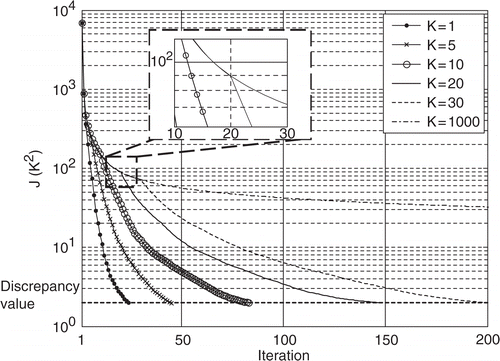
Figure 21. Computation time of the estimation of the interface (a) with the HSC (1), σ = 0.1 K, as a function of the update parameter K.
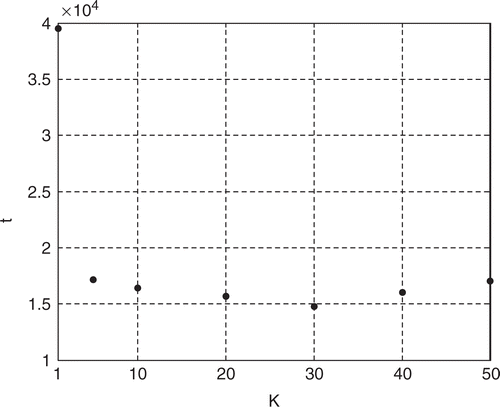
Figure 23. Interface thermal resistance distribution (K m2 W−1) searched to illustrate the refinement method.
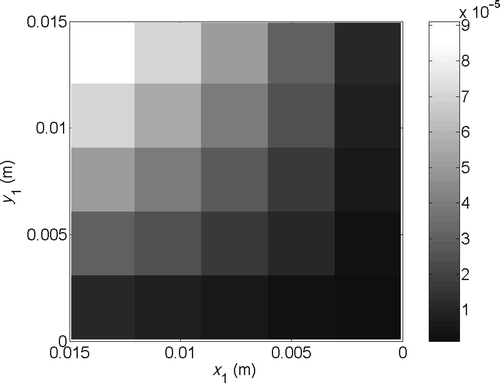
Figure 24. Estimated distributions (K m2 W−1) for the first six refinement levels with the HSC (1), σ = 0.1 K and .
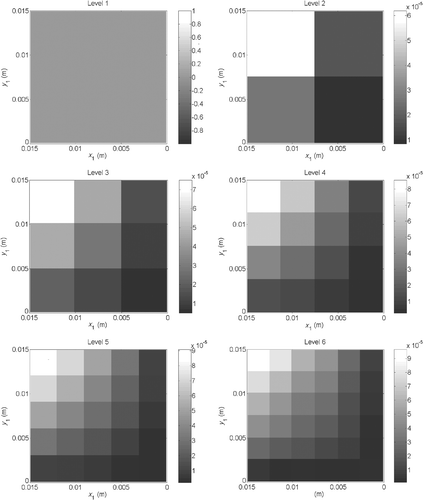
Figure 25. Evolution of the functional J for the first six refinement levels with the HSC (1), σ = 0.1 K and .
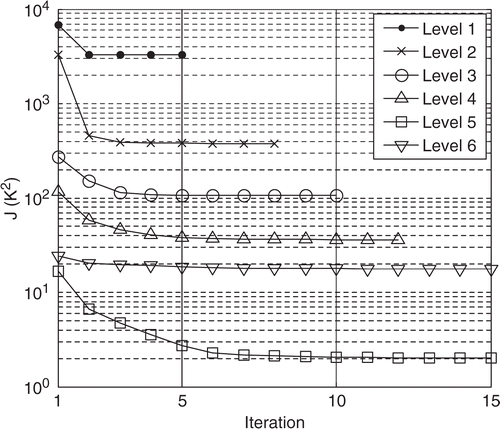